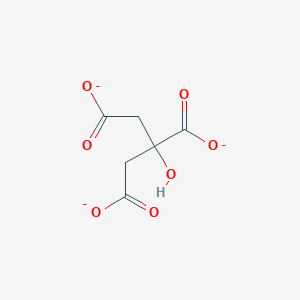
Citrato
Descripción general
Descripción
Citrate, or citric acid, is a weak organic acid that naturally occurs in citrus fruits. It is a key intermediate in the citric acid cycle (Krebs cycle), which is essential for the metabolic processes of all aerobic organisms . Citrate plays a crucial role in cellular energy production and is widely used in various industries due to its chelating properties.
Aplicaciones Científicas De Investigación
Citrate has numerous applications in scientific research, including:
Chemistry: Used as a buffer in chemical reactions and as a chelating agent to bind metal ions.
Biology: Plays a vital role in cellular metabolism and energy production.
Medicine: Used as an anticoagulant in blood transfusions and as a component in oral rehydration solutions.
Industry: Employed in food and beverage industries as a flavoring agent and preservative.
Mecanismo De Acción
Target of Action
Citrate primarily targets the Solute Carrier Family 13 Member 5 (SLC13A5) . SLC13A5 is a sodium-coupled citrate co-transporter that mediates the entry of extracellular citrate into the cytosol . Inhibition of SLC13A5 has been proposed as a target for reducing the progression of kidney disease . Citrate also directly inhibits the main regulators of glycolysis, phosphofructokinase-1 (PFK1) and phosphofructokinase-2 (PFK2) .
Mode of Action
Citrate works by chelating free calcium ions, preventing them from forming a complex with tissue factor and coagulation factor VIIa to promote the activation of coagulation factor X . This inhibits the extrinsic initiation of the coagulation cascade . Citrate also neutralizes acid in the stomach and urine, raising the pH .
Biochemical Pathways
Citrate is involved in numerous metabolic pathways, including the tricarboxylic acid (TCA) cycle, glucose, and lipid metabolism as well as post-translational modification and metal chelating . The principal competing pathway in wild-type Escherichia coli for acetyl-CoA is mediated by citrate synthase (coded by gltA), which directs over 60% of the acetyl-CoA into the tricarboxylic acid cycle .
Pharmacokinetics (ADME Properties)
The absorption, distribution, metabolism, elimination, and toxicity (ADMET) properties of citrate are crucial aspects of its bioavailability . .
Result of Action
Citrate plays a central role in cancer cells’ metabolism and regulation . Derived from mitochondrial synthesis and/or carboxylation of α-ketoglutarate, it is cleaved by ATP-citrate lyase into acetyl-CoA and oxaloacetate . The rapid turnover of these molecules in proliferative cancer cells maintains a low level of citrate, precluding its retro-inhibition on glycolytic enzymes .
Action Environment
Environmental factors such as the plant source:sink ratio, mineral fertilization, water supply, and temperature are the agro-environmental factors that have the most impact on fruit acidity . .
Análisis Bioquímico
Biochemical Properties
Citrate plays a crucial role in biochemical reactions. It interacts with various enzymes, proteins, and other biomolecules. For instance, bacteria that can grow on citrate medium produce an enzyme, citrate-permease, capable of converting citrate to pyruvate . Pyruvate can then enter the organism’s metabolic cycle for the production of energy .
Cellular Effects
Citrate has significant effects on various types of cells and cellular processes. It influences cell function, including impact on cell signaling pathways, gene expression, and cellular metabolism. For example, the methods for quantifying citrate concentration in cells can enable the study of the molecular mechanisms of citrate-related biological processes and diseases .
Molecular Mechanism
Citrate exerts its effects at the molecular level through various mechanisms. These include binding interactions with biomolecules, enzyme inhibition or activation, and changes in gene expression. For instance, when bacteria metabolize citrate, the ammonium salts are broken down to ammonia, which increases alkalinity .
Métodos De Preparación
Synthetic Routes and Reaction Conditions: Citrate can be synthesized through the fermentation of carbohydrates using microorganisms such as Aspergillus niger. The process involves the following steps:
Fermentation: Carbohydrates like glucose are fermented by Aspergillus niger to produce citric acid.
Precipitation: Calcium hydroxide is added to the fermentation broth to precipitate calcium citrate.
Acidification: Sulfuric acid is used to convert calcium citrate to citric acid.
Industrial Production Methods: The industrial production of citrate primarily involves submerged fermentation using Aspergillus niger. This method is preferred due to its high yield and efficiency. The process includes:
Preparation of Fermentation Medium: A nutrient-rich medium containing carbohydrates, nitrogen sources, and minerals is prepared.
Inoculation and Fermentation: The medium is inoculated with Aspergillus niger spores and fermented under controlled conditions.
Recovery and Purification: Citric acid is recovered from the fermentation broth through filtration, precipitation, and acidification.
Análisis De Reacciones Químicas
Types of Reactions: Citrate undergoes various chemical reactions, including:
Oxidation: Citrate can be oxidized to form carbon dioxide and water in the citric acid cycle.
Reduction: Citrate can be reduced to form isocitrate in the presence of enzymes.
Substitution: Citrate can undergo substitution reactions to form different citrate salts, such as sodium citrate and calcium citrate.
Common Reagents and Conditions:
Oxidation: Enzymes like citrate synthase and aconitase are involved in the oxidation of citrate in the citric acid cycle.
Reduction: Enzymes like isocitrate dehydrogenase facilitate the reduction of citrate.
Substitution: Reagents like sodium hydroxide and calcium hydroxide are used to form citrate salts.
Major Products:
Carbon dioxide and water: from oxidation.
Isocitrate: from reduction.
Sodium citrate and calcium citrate: from substitution.
Comparación Con Compuestos Similares
Malate: Another intermediate in the citric acid cycle, involved in the conversion of fumarate to oxaloacetate.
Oxaloacetate: A key intermediate in the citric acid cycle, involved in the condensation with acetyl-CoA to form citrate.
Isocitrate: An isomer of citrate, formed during the citric acid cycle.
Uniqueness of Citrate: Citrate is unique due to its ability to chelate metal ions and its central role in the citric acid cycle. Its versatility in various chemical reactions and applications in multiple industries further distinguishes it from other similar compounds .
Propiedades
IUPAC Name |
2-hydroxypropane-1,2,3-tricarboxylate | |
---|---|---|
Source | PubChem | |
URL | https://pubchem.ncbi.nlm.nih.gov | |
Description | Data deposited in or computed by PubChem | |
InChI |
InChI=1S/C6H8O7/c7-3(8)1-6(13,5(11)12)2-4(9)10/h13H,1-2H2,(H,7,8)(H,9,10)(H,11,12)/p-3 | |
Source | PubChem | |
URL | https://pubchem.ncbi.nlm.nih.gov | |
Description | Data deposited in or computed by PubChem | |
InChI Key |
KRKNYBCHXYNGOX-UHFFFAOYSA-K | |
Source | PubChem | |
URL | https://pubchem.ncbi.nlm.nih.gov | |
Description | Data deposited in or computed by PubChem | |
Canonical SMILES |
C(C(=O)[O-])C(CC(=O)[O-])(C(=O)[O-])O | |
Source | PubChem | |
URL | https://pubchem.ncbi.nlm.nih.gov | |
Description | Data deposited in or computed by PubChem | |
Molecular Formula |
C6H5O7-3 | |
Source | PubChem | |
URL | https://pubchem.ncbi.nlm.nih.gov | |
Description | Data deposited in or computed by PubChem | |
DSSTOX Substance ID |
DTXSID30155037 | |
Record name | Citrate | |
Source | EPA DSSTox | |
URL | https://comptox.epa.gov/dashboard/DTXSID30155037 | |
Description | DSSTox provides a high quality public chemistry resource for supporting improved predictive toxicology. | |
Molecular Weight |
189.10 g/mol | |
Source | PubChem | |
URL | https://pubchem.ncbi.nlm.nih.gov | |
Description | Data deposited in or computed by PubChem | |
Physical Description |
Tetrahydrate: White deliquescent solid; [Merck Index] | |
Record name | Lithium citrate | |
Source | Haz-Map, Information on Hazardous Chemicals and Occupational Diseases | |
URL | https://haz-map.com/Agents/14721 | |
Description | Haz-Map® is an occupational health database designed for health and safety professionals and for consumers seeking information about the adverse effects of workplace exposures to chemical and biological agents. | |
Explanation | Copyright (c) 2022 Haz-Map(R). All rights reserved. Unless otherwise indicated, all materials from Haz-Map are copyrighted by Haz-Map(R). No part of these materials, either text or image may be used for any purpose other than for personal use. Therefore, reproduction, modification, storage in a retrieval system or retransmission, in any form or by any means, electronic, mechanical or otherwise, for reasons other than personal use, is strictly prohibited without prior written permission. | |
CAS No. |
126-44-3 | |
Record name | Citrate(3-) | |
Source | CAS Common Chemistry | |
URL | https://commonchemistry.cas.org/detail?cas_rn=126-44-3 | |
Description | CAS Common Chemistry is an open community resource for accessing chemical information. Nearly 500,000 chemical substances from CAS REGISTRY cover areas of community interest, including common and frequently regulated chemicals, and those relevant to high school and undergraduate chemistry classes. This chemical information, curated by our expert scientists, is provided in alignment with our mission as a division of the American Chemical Society. | |
Explanation | The data from CAS Common Chemistry is provided under a CC-BY-NC 4.0 license, unless otherwise stated. | |
Record name | Citrate | |
Source | ChemIDplus | |
URL | https://pubchem.ncbi.nlm.nih.gov/substance/?source=chemidplus&sourceid=0000126443 | |
Description | ChemIDplus is a free, web search system that provides access to the structure and nomenclature authority files used for the identification of chemical substances cited in National Library of Medicine (NLM) databases, including the TOXNET system. | |
Record name | Citrate | |
Source | EPA DSSTox | |
URL | https://comptox.epa.gov/dashboard/DTXSID30155037 | |
Description | DSSTox provides a high quality public chemistry resource for supporting improved predictive toxicology. | |
Record name | CITRATE ION | |
Source | FDA Global Substance Registration System (GSRS) | |
URL | https://gsrs.ncats.nih.gov/ginas/app/beta/substances/664CCH53PI | |
Description | The FDA Global Substance Registration System (GSRS) enables the efficient and accurate exchange of information on what substances are in regulated products. Instead of relying on names, which vary across regulatory domains, countries, and regions, the GSRS knowledge base makes it possible for substances to be defined by standardized, scientific descriptions. | |
Explanation | Unless otherwise noted, the contents of the FDA website (www.fda.gov), both text and graphics, are not copyrighted. They are in the public domain and may be republished, reprinted and otherwise used freely by anyone without the need to obtain permission from FDA. Credit to the U.S. Food and Drug Administration as the source is appreciated but not required. | |
Synthesis routes and methods I
Procedure details
Synthesis routes and methods II
Procedure details
Synthesis routes and methods III
Procedure details
Retrosynthesis Analysis
AI-Powered Synthesis Planning: Our tool employs the Template_relevance Pistachio, Template_relevance Bkms_metabolic, Template_relevance Pistachio_ringbreaker, Template_relevance Reaxys, Template_relevance Reaxys_biocatalysis model, leveraging a vast database of chemical reactions to predict feasible synthetic routes.
One-Step Synthesis Focus: Specifically designed for one-step synthesis, it provides concise and direct routes for your target compounds, streamlining the synthesis process.
Accurate Predictions: Utilizing the extensive PISTACHIO, BKMS_METABOLIC, PISTACHIO_RINGBREAKER, REAXYS, REAXYS_BIOCATALYSIS database, our tool offers high-accuracy predictions, reflecting the latest in chemical research and data.
Strategy Settings
Precursor scoring | Relevance Heuristic |
---|---|
Min. plausibility | 0.01 |
Model | Template_relevance |
Template Set | Pistachio/Bkms_metabolic/Pistachio_ringbreaker/Reaxys/Reaxys_biocatalysis |
Top-N result to add to graph | 6 |
Feasible Synthetic Routes
A: Tripotassium citrate can be used as a substitute for sodium bicarbonate and potassium chloride in oral rehydration solutions for treating diarrhea and acidosis. Studies have shown its effectiveness in correcting dehydration and acidosis, particularly in children. [] This formulation also benefits patients with hypokalemia by improving serum potassium levels without causing hyperkalemia. []
A: Citrate anions in solution readily adsorb onto the hydrated aluminum oxide surface formed on aluminum electrodes. [] This adsorption, being competitive and reversible, influences the dissolution kinetics of aluminum. [] The presence of citrate ions, along with fluoride ions, can shift the dissolution process from surface-controlled to a mixed (transport- and surface-controlled) mechanism. [] This characteristic proves valuable in electrochemical studies, allowing researchers to discern between transport-controlled and surface-controlled processes on the aluminum electrode surface. []
A: Studies on potato starch show that esterification with citric acid, followed by acid hydrolysis, leads to the formation of starch citrate hydrolysates with modified properties. [] The degree of substitution with citrate increases with the temperature used during esterification. [] Conversely, higher esterification temperatures result in lower water absorption and solubility of the starch esters. [] This modification technique allows for tailoring starch's functional properties for specific applications.
A: Citrate, with its three carboxyl groups, plays a crucial role in energy metabolism. As a key component of the citric acid cycle, it is involved in the oxidation of acetyl-CoA, ultimately leading to ATP production. [] Citrate's structure also allows it to act as a chelating agent, binding to metal ions like calcium. This property is essential in preventing the formation of kidney stones by inhibiting calcium oxalate crystallization. []
A: In mammalian cells, citrate transport is crucial for various metabolic processes. Studies on guinea pig hearts revealed a citrate transporting system in heart mitochondria, albeit with lower activity than in liver mitochondria. [] This system facilitates the exchange of citrate with other metabolites like L-malate and phosphoenolpyruvate, impacting energy metabolism in the heart. []
A: Research indicates that the timing of citrate addition during the synthesis of zinc oxide nanostructures significantly influences the final morphology. When citrates are added before the formation of the Zn(OH)2 precursor, well-dispersed ZnO plates with highly exposed (001) facets are formed. [] Conversely, adding citrates after Zn(OH)2 precursor formation leads to ZnO nanoparticles. [] This suggests that the interaction of citrates with the precursor plays a vital role in directing the growth of ZnO plates with specific facet exposure, which is crucial for applications like photocatalysis. []
A: Research suggests that incorporating citrate into drug delivery systems, like calcium carbonate nanoparticles carrying citrate, shows promise in treating metabolic acidosis. [] These nanoparticles, with their small size (50-80 nm) and negative surface charge, exhibit low cytotoxicity and demonstrate effective cellular uptake in acidic environments. [] While they do not directly alkalinize the cellular environment or elevate bicarbonate levels, they exhibit antioxidant properties and protect against acidosis-induced cell death. [] This approach offers a potential alternative to traditional treatments, warranting further investigation into their systemic effects. []
A: Citrate synthase, a key enzyme in the citric acid cycle, utilizes acetyl-CoA and oxaloacetate to form citrate. Interestingly, research on sea anemones revealed that citrate synthase from these osmoconformers exhibits allosteric kinetics, unlike those from osmoregulators, which show hyperbolic kinetics. [] This difference suggests an adaptation strategy for maintaining metabolic function in fluctuating salinity environments, highlighting the diverse regulatory mechanisms of citrate synthase across different species. []
A: Yes, using solid dispersion techniques with citrate as a carrier can enhance the dissolution rate of poorly soluble drugs like Mosapride Citrate. [] Studies show that a solid dispersion of Mosapride Citrate with providone K30 as the carrier significantly improves its dissolution rate. [] At a ratio of 2:1 or higher (providone K30:Mosapride Citrate), near-complete drug dissolution is achieved within 5 minutes. [] This approach addresses the challenge of poor drug solubility, potentially improving its bioavailability. []
Descargo de responsabilidad e información sobre productos de investigación in vitro
Tenga en cuenta que todos los artículos e información de productos presentados en BenchChem están destinados únicamente con fines informativos. Los productos disponibles para la compra en BenchChem están diseñados específicamente para estudios in vitro, que se realizan fuera de organismos vivos. Los estudios in vitro, derivados del término latino "in vidrio", involucran experimentos realizados en entornos de laboratorio controlados utilizando células o tejidos. Es importante tener en cuenta que estos productos no se clasifican como medicamentos y no han recibido la aprobación de la FDA para la prevención, tratamiento o cura de ninguna condición médica, dolencia o enfermedad. Debemos enfatizar que cualquier forma de introducción corporal de estos productos en humanos o animales está estrictamente prohibida por ley. Es esencial adherirse a estas pautas para garantizar el cumplimiento de los estándares legales y éticos en la investigación y experimentación.