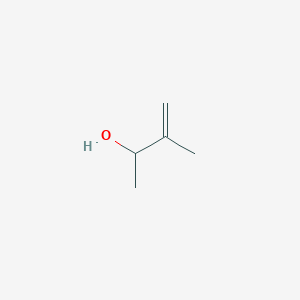
3-Methyl-3-buten-2-ol
Descripción general
Descripción
3-Methyl-3-buten-2-ol is a biogenic volatile organic compound (BVOC) emitted by pine trees and is a potential precursor of atmospheric secondary organic aerosol in forested regions . It is also an important component of the pheromone of the bark beetle Ips typographus . Its linear formula is C5H10O .
Synthesis Analysis
In a typical synthesis process, Pluronic F127 is dissolved in anhydrous ethanol or a mixture of ethanol and butanol and heated under vigorous stirring at 333 K for 10 min, followed by the addition of anhydrous ethanol suspension of colloidal PdZn nanoparticles .
Molecular Structure Analysis
The molecular structure of 3-Methyl-3-buten-2-ol is represented by the linear formula C5H10O . The molecular weight is 86.135 .
Chemical Reactions Analysis
The photoionization and dissociation processes of 3-Methyl-3-buten-2-ol in the energy range of 8.0–15.0 eV have been studied by tunable VUV synchrotron radiation coupled with a time-of-flight mass spectrometer . The direct elimination of CH3 is the most dominant channel and produces the resonance-stabilized radical cation .
Physical And Chemical Properties Analysis
3-Methyl-3-buten-2-ol has a vapor pressure of 51 mmHg at 25 °C, a refractive index n20/D of 1.416 (lit.), a boiling point of 98-99 °C (lit.), and a density of 0.824 g/mL at 25 °C (lit.) .
Aplicaciones Científicas De Investigación
Pyrolysis and Oxidation Studies
3-Methyl-3-buten-2-ol has been used in the study of pyrolysis and oxidation . The reactivity of unsaturated alcohols with a C=C double bond in the β- and γ-positions to the hydroxyl group is not well established. The pyrolysis and oxidation of 3-methyl-3-butenol have been studied, providing valuable insights into the reactivity of unsaturated alcohols .
Kinetic Modeling
This compound has been used in kinetic modeling studies . A kinetic model has been developed using the automatic network generation tool “Genesys”. Several important rate coefficients are obtained from new quantum chemical calculations .
Hydrogenation Reactions
3-Methyl-3-buten-2-ol has been used in the study of hydrogenation reactions . The efficiency of PdZn/Ti x M 1−x O 2±y (M = Ce, Zr, Zn) in the hydrogenation of 2-methyl-3-butyn 2-ol was studied with an emphasis on the stability of the catalyst during the reaction .
Development of Catalytic Coatings
The development of active, selective, and stable multicrystalline catalytic coatings on the inner surface of microcapillary reactors addresses environmental problems of fine organic synthesis . In this context, 3-Methyl-3-buten-2-ol has been used to study the formation of these coatings .
Pheromone Component
3-Methyl-3-buten-2-ol is reported to be an important component of the pheromone of the bark beetle Ips typographus . This highlights its potential use in ecological studies and pest management strategies.
Gas-Phase Reaction Studies
This compound has been used to study the kinetics of the gas-phase reaction of OH radicals with 3-methyl-2-butenal . This research can help understand the behavior of these compounds in the atmosphere.
Mecanismo De Acción
Target of Action
3-Methyl-3-buten-2-ol, also known as 3-methylbut-3-en-2-ol, is a biogenic volatile organic compound (BVOC) emitted by pine trees . It is an important component of the pheromone of the bark beetle Ips typographus .
Mode of Action
It is known that the compound can undergo reactions such as oxidation and pyrolysis . In the oxidation process, isoprenol consumption is dominated by a unimolecular reaction to formaldehyde and isobutene .
Biochemical Pathways
It has been suggested that the compound can be involved in the formation of secondary organic aerosols (soa) in the atmosphere . This process involves the uptake of 2-methyl-3-buten-2-ol into aqueous mixed solutions of sulfuric acid and hydrogen peroxide .
Pharmacokinetics
It is known that the compound can undergo pyrolysis and oxidation reactions .
Result of Action
It is known that the compound can undergo reactions to form products such as formaldehyde and isobutene .
Action Environment
3-Methyl-3-buten-2-ol is a biogenic volatile organic compound (BVOC) that is emitted in large quantities by pine trees . Its action can be influenced by environmental factors such as temperature and pressure . For example, experiments on the pyrolysis and oxidation of the compound were performed at temperatures ranging from 500 to 1100 K and a pressure of 0.107 MPa .
Safety and Hazards
3-Methyl-3-buten-2-ol is flammable and harmful if inhaled. It may cause respiratory irritation . It is recommended to wear personal protective equipment/face protection, ensure adequate ventilation, avoid ingestion and inhalation, and keep away from open flames, hot surfaces, and sources of ignition .
Direcciones Futuras
Propiedades
IUPAC Name |
3-methylbut-3-en-2-ol | |
---|---|---|
Source | PubChem | |
URL | https://pubchem.ncbi.nlm.nih.gov | |
Description | Data deposited in or computed by PubChem | |
InChI |
InChI=1S/C5H10O/c1-4(2)5(3)6/h5-6H,1H2,2-3H3 | |
Source | PubChem | |
URL | https://pubchem.ncbi.nlm.nih.gov | |
Description | Data deposited in or computed by PubChem | |
InChI Key |
JEYLKNVLTAPJAF-UHFFFAOYSA-N | |
Source | PubChem | |
URL | https://pubchem.ncbi.nlm.nih.gov | |
Description | Data deposited in or computed by PubChem | |
Canonical SMILES |
CC(C(=C)C)O | |
Source | PubChem | |
URL | https://pubchem.ncbi.nlm.nih.gov | |
Description | Data deposited in or computed by PubChem | |
Molecular Formula |
C5H10O | |
Source | PubChem | |
URL | https://pubchem.ncbi.nlm.nih.gov | |
Description | Data deposited in or computed by PubChem | |
DSSTOX Substance ID |
DTXSID30871205 | |
Record name | 3-Methyl-3-buten-2-ol | |
Source | EPA DSSTox | |
URL | https://comptox.epa.gov/dashboard/DTXSID30871205 | |
Description | DSSTox provides a high quality public chemistry resource for supporting improved predictive toxicology. | |
Molecular Weight |
86.13 g/mol | |
Source | PubChem | |
URL | https://pubchem.ncbi.nlm.nih.gov | |
Description | Data deposited in or computed by PubChem | |
Product Name |
3-Methyl-3-buten-2-ol | |
CAS RN |
10473-14-0 | |
Record name | 3-Methyl-3-buten-2-ol | |
Source | CAS Common Chemistry | |
URL | https://commonchemistry.cas.org/detail?cas_rn=10473-14-0 | |
Description | CAS Common Chemistry is an open community resource for accessing chemical information. Nearly 500,000 chemical substances from CAS REGISTRY cover areas of community interest, including common and frequently regulated chemicals, and those relevant to high school and undergraduate chemistry classes. This chemical information, curated by our expert scientists, is provided in alignment with our mission as a division of the American Chemical Society. | |
Explanation | The data from CAS Common Chemistry is provided under a CC-BY-NC 4.0 license, unless otherwise stated. | |
Record name | 3-Methyl-3-buten-2-ol | |
Source | ChemIDplus | |
URL | https://pubchem.ncbi.nlm.nih.gov/substance/?source=chemidplus&sourceid=0010473140 | |
Description | ChemIDplus is a free, web search system that provides access to the structure and nomenclature authority files used for the identification of chemical substances cited in National Library of Medicine (NLM) databases, including the TOXNET system. | |
Record name | 3-Methyl-3-buten-2-ol | |
Source | EPA DSSTox | |
URL | https://comptox.epa.gov/dashboard/DTXSID30871205 | |
Description | DSSTox provides a high quality public chemistry resource for supporting improved predictive toxicology. | |
Record name | 3-methyl-3-buten-2-ol | |
Source | European Chemicals Agency (ECHA) | |
URL | https://echa.europa.eu/substance-information/-/substanceinfo/100.030.864 | |
Description | The European Chemicals Agency (ECHA) is an agency of the European Union which is the driving force among regulatory authorities in implementing the EU's groundbreaking chemicals legislation for the benefit of human health and the environment as well as for innovation and competitiveness. | |
Explanation | Use of the information, documents and data from the ECHA website is subject to the terms and conditions of this Legal Notice, and subject to other binding limitations provided for under applicable law, the information, documents and data made available on the ECHA website may be reproduced, distributed and/or used, totally or in part, for non-commercial purposes provided that ECHA is acknowledged as the source: "Source: European Chemicals Agency, http://echa.europa.eu/". Such acknowledgement must be included in each copy of the material. ECHA permits and encourages organisations and individuals to create links to the ECHA website under the following cumulative conditions: Links can only be made to webpages that provide a link to the Legal Notice page. | |
Record name | 3-METHYL-3-BUTEN-2-OL | |
Source | FDA Global Substance Registration System (GSRS) | |
URL | https://gsrs.ncats.nih.gov/ginas/app/beta/substances/KG8M3UNR9E | |
Description | The FDA Global Substance Registration System (GSRS) enables the efficient and accurate exchange of information on what substances are in regulated products. Instead of relying on names, which vary across regulatory domains, countries, and regions, the GSRS knowledge base makes it possible for substances to be defined by standardized, scientific descriptions. | |
Explanation | Unless otherwise noted, the contents of the FDA website (www.fda.gov), both text and graphics, are not copyrighted. They are in the public domain and may be republished, reprinted and otherwise used freely by anyone without the need to obtain permission from FDA. Credit to the U.S. Food and Drug Administration as the source is appreciated but not required. | |
Synthesis routes and methods
Procedure details
Retrosynthesis Analysis
AI-Powered Synthesis Planning: Our tool employs the Template_relevance Pistachio, Template_relevance Bkms_metabolic, Template_relevance Pistachio_ringbreaker, Template_relevance Reaxys, Template_relevance Reaxys_biocatalysis model, leveraging a vast database of chemical reactions to predict feasible synthetic routes.
One-Step Synthesis Focus: Specifically designed for one-step synthesis, it provides concise and direct routes for your target compounds, streamlining the synthesis process.
Accurate Predictions: Utilizing the extensive PISTACHIO, BKMS_METABOLIC, PISTACHIO_RINGBREAKER, REAXYS, REAXYS_BIOCATALYSIS database, our tool offers high-accuracy predictions, reflecting the latest in chemical research and data.
Strategy Settings
Precursor scoring | Relevance Heuristic |
---|---|
Min. plausibility | 0.01 |
Model | Template_relevance |
Template Set | Pistachio/Bkms_metabolic/Pistachio_ringbreaker/Reaxys/Reaxys_biocatalysis |
Top-N result to add to graph | 6 |
Feasible Synthetic Routes
Descargo de responsabilidad e información sobre productos de investigación in vitro
Tenga en cuenta que todos los artículos e información de productos presentados en BenchChem están destinados únicamente con fines informativos. Los productos disponibles para la compra en BenchChem están diseñados específicamente para estudios in vitro, que se realizan fuera de organismos vivos. Los estudios in vitro, derivados del término latino "in vidrio", involucran experimentos realizados en entornos de laboratorio controlados utilizando células o tejidos. Es importante tener en cuenta que estos productos no se clasifican como medicamentos y no han recibido la aprobación de la FDA para la prevención, tratamiento o cura de ninguna condición médica, dolencia o enfermedad. Debemos enfatizar que cualquier forma de introducción corporal de estos productos en humanos o animales está estrictamente prohibida por ley. Es esencial adherirse a estas pautas para garantizar el cumplimiento de los estándares legales y éticos en la investigación y experimentación.