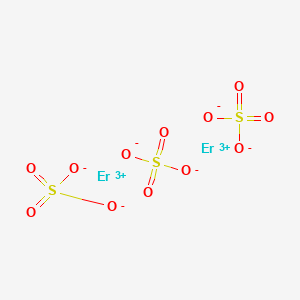
Erbium sulfate
Descripción general
Descripción
Erbium sulfate (Er₂(SO₄)₃), commonly encountered as the octahydrate (Er₂(SO₄)₃·8H₂O), is a trivalent lanthanide sulfate compound with a molecular weight of 766.82 g/mol and CAS number 10031-52-4 . It is commercially available in high purity (≥99.9%) and is utilized in industrial and research applications, including laser materials, catalysts, and spectroscopic studies .
Métodos De Preparación
Synthetic Routes and Reaction Conditions: Erbium sulfate can be synthesized through the reaction of erbium oxide (Er₂O₃) with sulfuric acid (H₂SO₄). The reaction typically involves dissolving erbium oxide in concentrated sulfuric acid, followed by evaporation of the solution to obtain this compound crystals. The reaction can be represented as follows: [ \text{Er}_2\text{O}_3 + 3\text{H}_2\text{SO}_4 \rightarrow \text{Er}_2(\text{SO}_4)_3 + 3\text{H}_2\text{O} ]
Industrial Production Methods: In industrial settings, this compound is produced by extracting erbium from minerals such as xenotime and monazite, which contain a mixture of rare earth elements. The extraction process involves several steps, including solvent extraction and ion-exchange methods, to isolate erbium. The isolated erbium is then reacted with sulfuric acid to produce this compound .
Análisis De Reacciones Químicas
Types of Reactions: Erbium sulfate undergoes various chemical reactions, including:
Oxidation and Reduction: this compound can participate in redox reactions, where erbium ions can change their oxidation state.
Substitution Reactions: this compound can undergo substitution reactions with other sulfate compounds, leading to the formation of different erbium salts.
Common Reagents and Conditions:
Oxidation: In the presence of strong oxidizing agents, this compound can be oxidized to form higher oxidation state compounds.
Reduction: Reducing agents can reduce this compound to lower oxidation state compounds.
Substitution: Reactions with other sulfate compounds or acids can lead to the substitution of sulfate ions.
Major Products Formed:
Erbium Oxide: Formed through the thermal decomposition of this compound.
Erbium Chloride: Formed through the reaction of this compound with hydrochloric acid.
Aplicaciones Científicas De Investigación
Industrial Applications
2.1. Catalysis
Erbium sulfate is utilized in catalytic processes, particularly in the petroleum industry. It serves as a catalyst in petrochemical cracking and automotive catalysts, enhancing the efficiency of hydrocarbon transformations . Additionally, it plays a role in environmental protection through catalytic processes that reduce harmful emissions.
2.2. Glass and Ceramics
In glass manufacturing, this compound acts as a colorant and is used to produce porcelain enamel glazes. Its high purity makes it suitable for optical applications where dopants are required for enhancing light transmission properties .
2.3. Rare Earth Fertilizers
This compound is also applied in the formulation of rare earth fertilizers, which are essential for improving soil quality and crop yields. The presence of erbium contributes to the overall nutrient profile of these fertilizers .
Medical Applications
3.1. Laser Therapy
One of the most notable medical applications of this compound is its use in laser treatments, specifically with erbium-doped yttrium aluminum garnet (YAG) lasers operating at 2940 nm. Research has demonstrated that this laser can stimulate hair growth by promoting the transition of hair follicles from the telogen to anagen phases . A study involving C57BL/6 mice showed significant increases in hair follicle counts and accelerated hair growth cycles following treatment with this laser .
3.2. Dermatological Treatments
Erbium lasers have been effectively used in dermatology for treating conditions such as facial angiofibromas associated with tuberous sclerosis. A case study reported successful outcomes using a combination of ablative laser therapy and medical treatments over two years .
Environmental Applications
4.1. Recovery of Rare Earth Elements
Recent studies have explored the recovery of rare earth elements (REEs) from industrial waste using sulfation-roasting-leaching processes that involve this compound. This method has shown promise in selectively recovering erbium from bottom ash, highlighting its potential role in sustainable practices within the recycling industry .
Summary of Properties and Applications
Property/Application | Description |
---|---|
Catalysis | Used in petrochemical cracking and automotive catalysts |
Glass Manufacturing | Acts as a colorant in glass and porcelain enamel glazes |
Fertilizers | Component in rare earth fertilizers for improved soil quality |
Laser Therapy | Stimulates hair growth using erbium-doped YAG lasers |
Dermatological Treatments | Effective in treating facial angiofibromas |
Environmental Recovery | Utilized in recovering rare earth elements from industrial waste |
Case Studies
- Hair Growth Stimulation: A study on C57BL/6 mice demonstrated that treatment with a 2940 nm erbium: YAG laser significantly increased hair follicle numbers and accelerated hair growth cycles compared to control groups .
- Dermatological Success: A 37-year-old female patient treated for facial angiofibromas showed marked improvement after a combination of ablative laser therapy and medication over two years .
- Sustainable Practices: Research on recovering REEs from bottom ash highlighted effective methods using this compound, showcasing its utility in promoting sustainability within industrial processes .
Mecanismo De Acción
Erbium sulfate can be compared with other similar compounds, such as:
Yttthis compound (Yb₂(SO₄)₃): Similar to this compound, yttthis compound is used in optical and luminescent applications. this compound is preferred in fiber-optic communication due to its specific emission wavelengths.
Dysprosium Sulfate (Dy₂(SO₄)₃): Dysprosium sulfate is another lanthanide sulfate with luminescent properties.
Comparación Con Compuestos Similares
Key Properties :
- Solubility : Erbium sulfate octahydrate exhibits a solubility of 16.00 g/100 mL at 20°C , significantly higher than europium sulfate octahydrate (2.56 g/100 mL) and dysprosium chromate (0.663 g/100 mL) .
- Thermal Behavior : this compound follows thermal decomposition patterns similar to dysprosium, holmium, and yttrium sulfates, forming intermediate oxides upon heating .
- Spectral Characteristics : The compound displays fine absorption bands in crystalline form, which broaden and shift with temperature changes .
Comparison with Similar Lanthanide Sulfates
Solubility and Structural Features
This compound’s solubility distinguishes it from other lanthanide sulfates (Table 1). For instance, europium sulfate octahydrate is far less soluble (2.56 g/100 mL), while erbium hydroxide (Er(OH)₃) is nearly insoluble (1.363×10⁻⁵ g/100 mL) . This high solubility facilitates its use in aqueous-phase applications like solvent sublation, where Er³+ recovery efficiency peaks at pH 8.0 with a distribution coefficient (K_r) of 2961.8 .
Table 1: Solubility of Selected Lanthanide Compounds (20°C)
Compound | Solubility (g/100 mL) |
---|---|
This compound octahydrate | 16.00 |
Europium sulfate octahydrate | 2.56 |
Dysprosium chromate | 0.663 |
Erbium hydroxide | 1.363×10⁻⁵ |
Thermal Decomposition Behavior
Thermogravimetric (TG-DTA) studies categorize lanthanide sulfates into three groups (Table 2):
Group 1 (Dy, Ho, Er, Y) : These compounds exhibit nearly identical decomposition profiles, forming intermediate oxysulfates (e.g., Er₂O(SO₄)₂) before converting to oxides (Er₂O₃) at high temperatures .
Group 2 (Tm, Yb, Lu) : These sulfates decompose at lower temperatures, producing distinct intermediates.
Tthis compound : Displays a unique decomposition pathway, suggesting divergent kinetic stability .
Table 2: Thermal Decomposition Groups of Heavy Lanthanide Sulfates
Group | Elements | Decomposition Characteristics |
---|---|---|
1 | Dy, Ho, Er, Y | Similar TG-DTA profiles; form oxysulfates |
2 | Tm, Yb, Lu | Lower decomposition temperatures |
3 | Tb | Unique pathway |
Solvent Sublation Efficiency
This compound demonstrates optimal recovery in solvent sublation systems at pH 8.0, with a recovery ratio (α) exceeding 95% when using sodium dodecyl sulfate (SDS) as a surfactant .
Spectral and Emission Behavior
This compound’s absorption spectra in crystalline form shift by ~10 Å between 100°C and 600°C due to dehydration, a phenomenon also observed in neodymium compounds . However, emission bands in erbium oxides are broader than those of neodymium, reflecting differences in 4f electron transitions .
Actividad Biológica
Erbium sulfate, particularly in its octahydrate form (Er₂(SO₄)₃·8H₂O), is a compound of erbium, a rare earth element that exhibits unique biological activities. This article delves into the biological activity of this compound, exploring its biochemical interactions, cellular effects, and potential applications in medicine and research.
Overview of this compound
This compound is primarily known for its role in materials science, but its interactions with biological systems have garnered interest due to its ability to form complexes with biomolecules. This property is leveraged in various biochemical applications, including proteomics and medical imaging.
Target Interactions
Erbium ions (Er³⁺) can bind to proteins and enzymes, influencing their structure and function. This binding can lead to enzyme inhibition or activation , depending on the specific biomolecule involved. For example, erbium ions can interact with calmodulin, a calcium-binding messenger protein, which may alter intracellular signaling pathways.
Biochemical Pathways
This compound participates in metabolic pathways related to sulfur metabolism. It can interact with enzymes involved in these pathways, affecting metabolic flux and levels of metabolites. The compound's stability and degradation over time are crucial factors influencing its biological effects.
Influence on Cell Signaling
Research indicates that this compound can modulate cell signaling pathways. For instance, it has been observed to alter gene expression and cellular metabolism through its interactions with signaling molecules. These effects suggest potential therapeutic applications in conditions where cell signaling is disrupted.
Dosage-Dependent Effects
The impact of this compound varies significantly with dosage. Low doses may have minimal effects on cellular function, while higher concentrations can induce notable changes in cell behavior and metabolism. In animal models, higher doses have been associated with increased triglyceride levels in the liver and alterations in hepatic enzyme leakage .
Hair Growth Stimulation via Er:YAG Laser
A notable study investigated the effects of a 2940 nm erbium: YAG laser on hair growth in C57BL/6 mice. The results showed that laser treatment significantly accelerated the transition from the telogen (resting) phase to the anagen (growth) phase of hair follicles. Histological analysis revealed an increase in hair follicle density and size in treated groups compared to controls .
Treatment Group | Hair Follicle Count (Anagen VI) | Time from Telogen to Anagen (Days) |
---|---|---|
Control | 19.5% | 12 |
Er:YAG Laser | 41.5% | 8 |
Minoxidil | 37.5% | 9 |
Combination (Laser + Minoxidil) | 44% | 7 |
This study highlights the potential of erbium compounds in dermatological applications, particularly for hair loss treatments.
Complexation with Biomolecules
In biochemical research, this compound has been utilized for its ability to form stable complexes with various ligands. This property is particularly useful for studying enzyme kinetics and protein interactions . For example, erbium ions can complex with EDTA and other chelating agents under ambient conditions, facilitating various biochemical assays.
Safety and Toxicity
While erbium itself does not play a significant biological role, its compounds can exhibit slight toxicity if ingested in large amounts. The average human consumption of erbium is about 1 mg per year, primarily stored in bones . However, studies indicate that ionic erbium behaves similarly to calcium ions and can influence metabolic processes without significant toxicity at low concentrations.
Q & A
Basic Research Questions
Q. What are the standard methods for synthesizing high-purity erbium sulfate, and how are purity levels validated?
- Methodology : this compound octahydrate (CAS 10031-52-4) is typically synthesized via slow evaporation of stoichiometric erbium oxide (Er₂O₃) dissolved in sulfuric acid under controlled temperature (80–90°C). Post-crystallization, purity is assessed using inductively coupled plasma optical emission spectrometry (ICP-OES) to quantify trace rare earth impurities (e.g., ≤553 ppm in 99.94% pure samples) and X-ray diffraction (XRD) to confirm crystallographic structure .
- Data Validation : Certificates of Analysis (CoA) should include parameters like appearance (pink crystals/powder), ICP confirmation of erbium content, and XRD consistency with reference databases .
Q. What safety protocols are critical when handling this compound in laboratory settings?
- Hazard Mitigation : Per safety data sheets (SDS), this compound is classified as H302 (harmful if swallowed), H315 (skin irritation), and H319 (eye irritation). Required precautions include:
- Use of PPE (gloves, goggles, lab coats).
- Engineering controls (fume hoods for powder handling).
- Immediate decontamination of spills with inert absorbents .
- Storage : Store in airtight containers away from incompatible materials (strong oxidizers, bases) at room temperature .
Q. Which spectroscopic techniques are most effective for characterizing this compound’s structural and optical properties?
- Structural Analysis : XRD is standard for verifying crystallinity and phase purity. For optical studies, UV-Vis-NIR spectroscopy identifies absorption bands (e.g., Er³⁺ transitions at 980 nm and 1530 nm), relevant for photonic applications .
- Advanced Techniques : Fourier-transform infrared spectroscopy (FTIR) confirms sulfate group vibrations (ν₃ SO₄²⁻ at ~1100 cm⁻¹) .
Advanced Research Questions
Q. How can researchers resolve contradictions in reported thermal decomposition pathways of this compound hydrates?
- Methodological Approach : Discrepancies in decomposition steps (e.g., octahydrate → tetrahydrate vs. direct anhydrous form) require controlled thermogravimetric analysis (TGA) under inert atmospheres. Key parameters:
- Heating rate (2–5°C/min) to avoid kinetic artifacts.
- Coupled mass spectrometry (MS) to identify evolved gases (e.g., H₂O, SO₃) .
- Case Study : Aladdin Scientific’s CoA for Er₂(SO₄)₃·8H₂O showed stability up to 100°C, with dehydration completing at 250°C .
Q. What experimental design considerations are essential for studying this compound’s efficacy in photonic or catalytic applications?
- Photonic Applications : For fiber-optic doping, optimize Er³⁺ concentration (0.1–1.0 wt%) using melt-quenching synthesis. Characterize emission lifetimes via time-resolved luminescence spectroscopy to assess quenching effects .
- Catalysis : In sulfate-assisted reactions (e.g., hydrolysis), control pH (2–4) and sulfate ion mobility using ionic conductivity measurements .
Q. How can computational modeling complement experimental studies of this compound’s electronic structure?
- Density Functional Theory (DFT) : Model Er³⁺ ligand-field splitting and charge-transfer transitions. Validate against experimental UV-Vis spectra to refine crystal field parameters .
- Molecular Dynamics (MD) : Simulate hydration shell dynamics around Er³⁺ ions to predict stability in aqueous systems .
Q. Data Contradiction and Reproducibility
Q. Why do reported solubility values for this compound vary across studies, and how can reproducibility be improved?
- Root Causes : Variability in hydration states (e.g., octahydrate vs. anhydrous) and pH-dependent solubility (higher solubility in acidic media).
- Standardization : Use IUPAC-recommended protocols:
Propiedades
Número CAS |
13478-49-4 |
---|---|
Fórmula molecular |
ErH2O4S |
Peso molecular |
265.34 g/mol |
Nombre IUPAC |
erbium;sulfuric acid |
InChI |
InChI=1S/Er.H2O4S/c;1-5(2,3)4/h;(H2,1,2,3,4) |
Clave InChI |
LWTVYECLHXQDIH-UHFFFAOYSA-N |
SMILES |
[O-]S(=O)(=O)[O-].[O-]S(=O)(=O)[O-].[O-]S(=O)(=O)[O-].[Er+3].[Er+3] |
SMILES canónico |
OS(=O)(=O)O.[Er] |
Key on ui other cas no. |
13478-49-4 |
Pictogramas |
Irritant |
Origen del producto |
United States |
Retrosynthesis Analysis
AI-Powered Synthesis Planning: Our tool employs the Template_relevance Pistachio, Template_relevance Bkms_metabolic, Template_relevance Pistachio_ringbreaker, Template_relevance Reaxys, Template_relevance Reaxys_biocatalysis model, leveraging a vast database of chemical reactions to predict feasible synthetic routes.
One-Step Synthesis Focus: Specifically designed for one-step synthesis, it provides concise and direct routes for your target compounds, streamlining the synthesis process.
Accurate Predictions: Utilizing the extensive PISTACHIO, BKMS_METABOLIC, PISTACHIO_RINGBREAKER, REAXYS, REAXYS_BIOCATALYSIS database, our tool offers high-accuracy predictions, reflecting the latest in chemical research and data.
Strategy Settings
Precursor scoring | Relevance Heuristic |
---|---|
Min. plausibility | 0.01 |
Model | Template_relevance |
Template Set | Pistachio/Bkms_metabolic/Pistachio_ringbreaker/Reaxys/Reaxys_biocatalysis |
Top-N result to add to graph | 6 |
Feasible Synthetic Routes
Descargo de responsabilidad e información sobre productos de investigación in vitro
Tenga en cuenta que todos los artículos e información de productos presentados en BenchChem están destinados únicamente con fines informativos. Los productos disponibles para la compra en BenchChem están diseñados específicamente para estudios in vitro, que se realizan fuera de organismos vivos. Los estudios in vitro, derivados del término latino "in vidrio", involucran experimentos realizados en entornos de laboratorio controlados utilizando células o tejidos. Es importante tener en cuenta que estos productos no se clasifican como medicamentos y no han recibido la aprobación de la FDA para la prevención, tratamiento o cura de ninguna condición médica, dolencia o enfermedad. Debemos enfatizar que cualquier forma de introducción corporal de estos productos en humanos o animales está estrictamente prohibida por ley. Es esencial adherirse a estas pautas para garantizar el cumplimiento de los estándares legales y éticos en la investigación y experimentación.