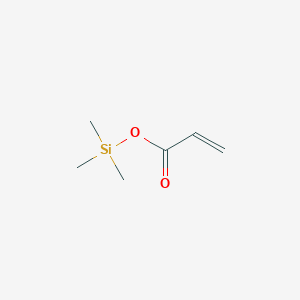
Trimethylsilyl acrylate
Descripción general
Descripción
Es un polímero formado cuando el ácido gálico, un monómero polifenólico, se esterifica y se une al grupo hidroxilo de un carbohidrato poliol como la glucosa . Los gallotaninos se encuentran ampliamente en la naturaleza, particularmente en plantas, frutas, vinos y tés . Son conocidos por sus propiedades astringentes y se han utilizado históricamente en el curtido del cuero .
Mecanismo De Acción
Los gallotaninos ejercen sus efectos a través de varios mecanismos:
Actividad antioxidante: Barren los radicales libres y reducen el estrés oxidativo.
Inhibición enzimática: Los gallotaninos inhiben enzimas como la lipooxigenasa y la ciclooxigenasa, que están involucradas en los procesos inflamatorios.
Unión a proteínas: Forman complejos con proteínas, lo que puede inhibir el crecimiento microbiano y la actividad enzimática.
Destinos moleculares y vías:
V ía JAK/STAT: Los gallotaninos modulan esta vía, que está involucrada en la proliferación celular y las respuestas inmunitarias.
V ía RAS/RAF/mTOR: Influyen en esta vía, que es crucial para el crecimiento y la supervivencia celular.
Análisis Bioquímico
Biochemical Properties
Trimethylsilyl acrylate plays a significant role in biochemical reactions due to its reactive nature. It interacts with various enzymes, proteins, and other biomolecules. For instance, it can undergo methoxycarbonylation in the presence of palladium catalysts, leading to the formation of valuable intermediates . The trimethylsilyl group in this compound can also interact with sugars and amino acids, facilitating the transfer of trimethylsilyl groups to these biomolecules . These interactions are crucial for the compound’s role in organic synthesis and polymer chemistry.
Cellular Effects
This compound has notable effects on various types of cells and cellular processes. It can influence cell function by interacting with cell membranes and other cellular components. Studies have shown that organosilicon compounds, including this compound, can be retained in or on cell membranes This retention can affect cell signaling pathways, gene expression, and cellular metabolism
Molecular Mechanism
The molecular mechanism of this compound involves its interactions at the molecular level. The trimethylsilyl group in the compound can act as a temporary protecting group during chemical synthesis, facilitating various chemical reactions . Additionally, this compound can undergo derivatization, making it more volatile and amenable to analysis by gas chromatography or mass spectrometry . These molecular interactions are essential for understanding the compound’s role in biochemical reactions and its applications in analytical chemistry.
Temporal Effects in Laboratory Settings
In laboratory settings, the effects of this compound can change over time. The compound’s stability and degradation are important factors to consider. This compound is known to be a flammable liquid with a flash point of -15°C . Its stability can be influenced by factors such as temperature and exposure to air. Long-term studies have shown that the compound can degrade over time, affecting its efficacy in biochemical reactions and its impact on cellular function.
Dosage Effects in Animal Models
The effects of this compound can vary with different dosages in animal models. Studies have shown that organosilicon compounds, including this compound, can have toxic or adverse effects at high doses . It is important to determine the threshold effects and safe dosage levels for the compound to avoid potential toxicity. Animal models are crucial for understanding the compound’s pharmacokinetics and its impact on biological systems.
Metabolic Pathways
This compound is involved in various metabolic pathways. The compound can undergo trimethylsilylation, a process that makes it more volatile and suitable for analysis by gas chromatography-mass spectrometry . This metabolic pathway is essential for the compound’s role in metabolite profiling and analytical chemistry. Additionally, this compound can interact with enzymes and cofactors, affecting metabolic flux and metabolite levels in biological systems.
Transport and Distribution
The transport and distribution of this compound within cells and tissues are influenced by its interactions with transporters and binding proteins. The compound’s trimethylsilyl group can facilitate its transport across cell membranes and its accumulation in specific cellular compartments . Understanding the transport and distribution of this compound is crucial for its applications in biotechnology and materials science.
Subcellular Localization
This compound’s subcellular localization is determined by its targeting signals and post-translational modifications. The compound can be directed to specific compartments or organelles within the cell, affecting its activity and function
Métodos De Preparación
Rutas sintéticas y condiciones de reacción: Los gallotaninos se pueden sintetizar mediante la esterificación del ácido gálico con glucosa. La reacción normalmente implica el uso de cloruro de ácido gálico protegido con bencilo como sustrato . Las condiciones de reacción que conducen a una alta selectividad anomérica para la síntesis de gallotaninos incluyen el uso de acetonitrilo como solvente .
Métodos de producción industrial: Industrialmente, los gallotaninos se extraen de fuentes vegetales como las agallas, el zumaque y las vainas de tara. El proceso de extracción implica el uso de solventes como agua o alcohol para extraer los taninos del material vegetal. El extracto se purifica luego para obtener gallotaninos .
Análisis De Reacciones Químicas
Tipos de reacciones: Los gallotaninos experimentan varias reacciones químicas, que incluyen:
Oxidación: Los gallotaninos se pueden oxidar para formar quinonas.
Hidrólisis: Se pueden hidrolizar para producir ácido gálico y glucosa.
Complejación: Los gallotaninos pueden formar complejos con proteínas e iones metálicos.
Reactivos y condiciones comunes:
Oxidación: Los agentes oxidantes comunes incluyen el permanganato de potasio y el peróxido de hidrógeno.
Hidrólisis: Se pueden usar condiciones ácidas o básicas para la hidrólisis.
Complejación: Los gallotaninos forman fácilmente complejos en condiciones neutras o ligeramente ácidas.
Productos principales:
Oxidación: Quinonas.
Hidrólisis: Ácido gálico y glucosa.
Complejación: Complejos proteína-tanino y complejos metal-tanino.
Aplicaciones Científicas De Investigación
Los gallotaninos tienen una amplia gama de aplicaciones de investigación científica:
Química: Utilizados como antioxidantes y en la síntesis de otros compuestos polifenólicos.
Biología: Estudiados por su papel en los mecanismos de defensa vegetal y las interacciones con los herbívoros.
Medicina: Investigados por sus propiedades anticancerígenas, antiinflamatorias y antimicrobianas.
Industria: Utilizados en la conservación de alimentos, el curtido del cuero y como aditivos en cosméticos.
Comparación Con Compuestos Similares
Los gallotaninos a menudo se comparan con otros taninos hidrolizables como los elagitaninos.
Compuestos similares:
Elagitaninos: Estos también son taninos hidrolizables, pero difieren en su estructura, conteniendo ácido hexahidroxidifenico en lugar de ácido gálico.
Taninos condensados: A diferencia de los taninos hidrolizables, los taninos condensados no se descomponen fácilmente y constan de unidades de flavonoides.
Singularidad de los gallotaninos:
Hidrolizabilidad: Los gallotaninos se hidrolizan fácilmente para producir ácido gálico y glucosa, lo que los distingue de los taninos condensados.
Bioactividad: Tienen propiedades bioactivas únicas, incluida una fuerte actividad antioxidante e inhibitoria de enzimas.
Los gallotaninos siguen siendo objeto de una amplia investigación debido a sus diversas aplicaciones y propiedades bioactivas significativas.
Propiedades
IUPAC Name |
trimethylsilyl prop-2-enoate | |
---|---|---|
Source | PubChem | |
URL | https://pubchem.ncbi.nlm.nih.gov | |
Description | Data deposited in or computed by PubChem | |
InChI |
InChI=1S/C6H12O2Si/c1-5-6(7)8-9(2,3)4/h5H,1H2,2-4H3 | |
Source | PubChem | |
URL | https://pubchem.ncbi.nlm.nih.gov | |
Description | Data deposited in or computed by PubChem | |
InChI Key |
OTYBJBJYBGWBHB-UHFFFAOYSA-N | |
Source | PubChem | |
URL | https://pubchem.ncbi.nlm.nih.gov | |
Description | Data deposited in or computed by PubChem | |
Canonical SMILES |
C[Si](C)(C)OC(=O)C=C | |
Source | PubChem | |
URL | https://pubchem.ncbi.nlm.nih.gov | |
Description | Data deposited in or computed by PubChem | |
Molecular Formula |
C6H12O2Si | |
Source | PubChem | |
URL | https://pubchem.ncbi.nlm.nih.gov | |
Description | Data deposited in or computed by PubChem | |
DSSTOX Substance ID |
DTXSID00339226 | |
Record name | Trimethylsilyl acrylate | |
Source | EPA DSSTox | |
URL | https://comptox.epa.gov/dashboard/DTXSID00339226 | |
Description | DSSTox provides a high quality public chemistry resource for supporting improved predictive toxicology. | |
Molecular Weight |
144.24 g/mol | |
Source | PubChem | |
URL | https://pubchem.ncbi.nlm.nih.gov | |
Description | Data deposited in or computed by PubChem | |
CAS No. |
13688-55-6 | |
Record name | Trimethylsilyl acrylate | |
Source | EPA DSSTox | |
URL | https://comptox.epa.gov/dashboard/DTXSID00339226 | |
Description | DSSTox provides a high quality public chemistry resource for supporting improved predictive toxicology. | |
Record name | Trimethylsilyl Acrylate (stabilized with BHT) | |
Source | European Chemicals Agency (ECHA) | |
URL | https://echa.europa.eu/information-on-chemicals | |
Description | The European Chemicals Agency (ECHA) is an agency of the European Union which is the driving force among regulatory authorities in implementing the EU's groundbreaking chemicals legislation for the benefit of human health and the environment as well as for innovation and competitiveness. | |
Explanation | Use of the information, documents and data from the ECHA website is subject to the terms and conditions of this Legal Notice, and subject to other binding limitations provided for under applicable law, the information, documents and data made available on the ECHA website may be reproduced, distributed and/or used, totally or in part, for non-commercial purposes provided that ECHA is acknowledged as the source: "Source: European Chemicals Agency, http://echa.europa.eu/". Such acknowledgement must be included in each copy of the material. ECHA permits and encourages organisations and individuals to create links to the ECHA website under the following cumulative conditions: Links can only be made to webpages that provide a link to the Legal Notice page. | |
Retrosynthesis Analysis
AI-Powered Synthesis Planning: Our tool employs the Template_relevance Pistachio, Template_relevance Bkms_metabolic, Template_relevance Pistachio_ringbreaker, Template_relevance Reaxys, Template_relevance Reaxys_biocatalysis model, leveraging a vast database of chemical reactions to predict feasible synthetic routes.
One-Step Synthesis Focus: Specifically designed for one-step synthesis, it provides concise and direct routes for your target compounds, streamlining the synthesis process.
Accurate Predictions: Utilizing the extensive PISTACHIO, BKMS_METABOLIC, PISTACHIO_RINGBREAKER, REAXYS, REAXYS_BIOCATALYSIS database, our tool offers high-accuracy predictions, reflecting the latest in chemical research and data.
Strategy Settings
Precursor scoring | Relevance Heuristic |
---|---|
Min. plausibility | 0.01 |
Model | Template_relevance |
Template Set | Pistachio/Bkms_metabolic/Pistachio_ringbreaker/Reaxys/Reaxys_biocatalysis |
Top-N result to add to graph | 6 |
Feasible Synthetic Routes
Q1: What are the typical reactions Trimethylsilyl acrylate undergoes and what makes it useful in organic synthesis?
A1: this compound is a versatile reagent in organic synthesis due to its participation in Michael additions and Peterson olefinations.
Q2: How does the presence of the Trimethylsilyl group influence the stereochemistry of reactions involving this compound?
A2: The Trimethylsilyl group plays a crucial role in directing the stereochemical outcome of reactions with this compound. For instance, Grignard reagents react with Methyl 2-(trimethylsilyl)acrylate to predominantly yield a single diastereomer of the 1:2 adduct after quenching with water [, ]. This high diastereoselectivity is attributed to tight chelation within the 1:2 adduct anions, a phenomenon influenced by the steric and electronic properties of the Trimethylsilyl group.
Q3: Can this compound be used to synthesize polymers with specific tacticity?
A3: Yes, this compound is a valuable monomer for synthesizing polymers with controlled stereochemistry. Notably, polymerization of this compound at low temperatures yields syndiotactic polymers []. This syndiotactic poly(this compound) can then be transformed into syndiotactic poly(methyl acrylate) [], highlighting a route to access polymers with desired properties influenced by tacticity.
Q4: Are there any spectroscopic techniques used to analyze the structure of polymers derived from this compound?
A4: Yes, Nuclear Magnetic Resonance (NMR) spectroscopy is a powerful tool for determining the stereoregularity of polymers produced from this compound and other acrylate monomers []. NMR analysis provides insights into the arrangement of monomer units within the polymer chain, allowing researchers to determine the degree of isotacticity, syndiotacticity, or atacticity. Additionally, infrared (IR) spectroscopy can provide an approximate estimation of stereoregularity in polymers like poly(methyl acrylate) [].
Q5: Beyond polymerization, are there other interesting applications of this compound in organic synthesis?
A5: Yes, this compound has proven valuable for synthesizing N-substituted β-Alanines [, ]. The Michael addition of various amines to this compound provides a direct route to these valuable building blocks commonly employed in medicinal chemistry and peptide research.
Descargo de responsabilidad e información sobre productos de investigación in vitro
Tenga en cuenta que todos los artículos e información de productos presentados en BenchChem están destinados únicamente con fines informativos. Los productos disponibles para la compra en BenchChem están diseñados específicamente para estudios in vitro, que se realizan fuera de organismos vivos. Los estudios in vitro, derivados del término latino "in vidrio", involucran experimentos realizados en entornos de laboratorio controlados utilizando células o tejidos. Es importante tener en cuenta que estos productos no se clasifican como medicamentos y no han recibido la aprobación de la FDA para la prevención, tratamiento o cura de ninguna condición médica, dolencia o enfermedad. Debemos enfatizar que cualquier forma de introducción corporal de estos productos en humanos o animales está estrictamente prohibida por ley. Es esencial adherirse a estas pautas para garantizar el cumplimiento de los estándares legales y éticos en la investigación y experimentación.