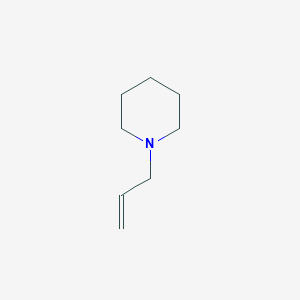
1-alilpiperidina
Descripción general
Descripción
1-allylpiperidine is an organic compound with the molecular formula C8H15N It is a derivative of piperidine, where an allyl group is attached to the nitrogen atom
Aplicaciones Científicas De Investigación
Medicinal Chemistry
1-Allylpiperidine serves as a key intermediate in the synthesis of various pharmaceutical compounds. Its structural properties allow it to be incorporated into drugs targeting different biological pathways.
- Synthesis of Bioactive Compounds : 1-Allylpiperidine is utilized in the synthesis of 4-chloro-2-piperidin-1-yl-quinazoline, which exhibits potential antitumor activity. The reaction is typically conducted at elevated temperatures (100°C), demonstrating its utility in producing biologically active molecules .
- Neuropharmacological Studies : Research has indicated that derivatives of 1-allylpiperidine can interact with neurotransmitter receptors, making them candidates for developing treatments for neurological disorders. For example, studies have focused on their effects on dopamine and serotonin receptors, which are crucial in managing conditions like depression and schizophrenia .
Organic Synthesis
1-Allylpiperidine is a valuable building block in organic synthesis due to its ability to undergo various chemical transformations.
- Allylation Reactions : It is commonly employed in allylation reactions to produce more complex piperidine derivatives. The use of palladium catalysts has been reported to yield high selectivity and efficiency in these reactions, allowing for the creation of diverse compounds with potential therapeutic applications .
- Synthesis of Polymers : In polymer science, 1-allylpiperidine derivatives have been used to create functionalized polymers that exhibit unique properties. For instance, poly(N,N-diallylazacycloalkane)s have been synthesized for use as anion-exchange membranes, showcasing the compound's versatility beyond small molecule synthesis .
Case Study 1: Synthesis of Piperidine Derivatives
A study detailed the synthesis of various piperidine derivatives using 1-allylpiperidine as a starting material. The research highlighted the effectiveness of different reaction conditions and catalysts in achieving high yields and selectivity. For instance, reactions involving allyl bromide and piperidine compounds demonstrated promising results in producing complex structures with potential biological activity .
Case Study 2: Polymer Applications
Another significant application was explored in the development of anion-exchange membranes using 1-allylpiperidine derivatives. These membranes were characterized for their thermal and alkaline stability, indicating their suitability for industrial applications such as fuel cells and water purification systems. The study emphasized the importance of functionalizing piperidine derivatives to enhance membrane performance .
Mecanismo De Acción
Target of Action
1-Allylpiperidine, also known as 1-(2-Propenyl)piperidine or N-Allylpiperidine, is a chemical compound that primarily targets the respiratory system . It is classified as a hazardous chemical by the 2012 OSHA Hazard Communication Standard .
Mode of Action
It is known to cause skin irritation, serious eye irritation, and may cause respiratory irritation . This suggests that it interacts with its targets, causing changes that lead to these effects.
Biochemical Pathways
Piperidine derivatives, which include 1-allylpiperidine, have been found to exhibit a wide variety of biological activities . They are utilized in different ways as anticancer, antiviral, antimalarial, antimicrobial, antifungal, antihypertension, analgesic, anti-inflammatory, anti-Alzheimer, antipsychotic, and/or anticoagulant agents .
Result of Action
Given its classification as a hazardous chemical and its known irritant effects, it can be inferred that the compound likely causes cellular damage or disruption in its target tissues .
Action Environment
The action, efficacy, and stability of 1-Allylpiperidine can be influenced by various environmental factors. For example, it is known to be flammable , suggesting that its stability and action can be affected by exposure to heat or open flames. Additionally, its solubility in water could impact its action and efficacy in aqueous environments .
Análisis Bioquímico
Biochemical Properties
It is known that 1-Allylpiperidine is a liquid at room temperature and has a boiling point of 151-152°C
Cellular Effects
Safety data sheets indicate that exposure to 1-Allylpiperidine may cause respiratory irritation and symptoms of overexposure may include headache, dizziness, tiredness, nausea, and vomiting
Temporal Effects in Laboratory Settings
It is known that 1-Allylpiperidine is stable at room temperature
Métodos De Preparación
Synthetic Routes and Reaction Conditions: 1-allylpiperidine can be synthesized through several methods. One common approach is the hydrosilylation reaction, where N-allylpiperidine is formed by reacting piperidine with allyl halides in the presence of a catalyst such as platinum(0) complex (Karstedt’s catalyst) under mild conditions . Another method involves the N-heterocyclization of primary amines with diols catalyzed by a Cp*Ir complex .
Industrial Production Methods: Industrial production of 1-allylpiperidine typically involves large-scale hydrosilylation reactions. The process is optimized for high yield and purity, often using continuous flow reactors to ensure consistent reaction conditions and efficient production .
Análisis De Reacciones Químicas
Types of Reactions: 1-allylpiperidine undergoes various chemical reactions, including:
Oxidation: It can be oxidized to form N-allylpiperidone.
Reduction: Reduction reactions can convert it into N-allylpiperidine derivatives with different functional groups.
Substitution: 1-allylpiperidine can participate in nucleophilic substitution reactions, where the allyl group can be replaced by other substituents.
Common Reagents and Conditions:
Oxidation: Common oxidizing agents include potassium permanganate and chromium trioxide.
Reduction: Reducing agents such as lithium aluminum hydride and sodium borohydride are used.
Substitution: Reagents like alkyl halides and acyl chlorides are employed in substitution reactions.
Major Products:
Oxidation: N-allylpiperidone.
Reduction: Various N-allylpiperidine derivatives.
Substitution: Substituted piperidines with different functional groups.
Comparación Con Compuestos Similares
N-Allylaniline: Similar in structure but with an aniline base instead of piperidine.
N-Allylcyclohexylamine: Contains a cyclohexylamine base instead of piperidine.
N-Allylpyrrolidine: A smaller ring structure compared to piperidine.
Uniqueness: 1-allylpiperidine is unique due to its six-membered ring structure, which provides stability and reactivity that are advantageous in various chemical reactions. Its ability to undergo diverse transformations makes it a valuable compound in synthetic organic chemistry.
Actividad Biológica
1-Allylpiperidine, an organic compound with the molecular formula C8H15N, is a derivative of piperidine characterized by the presence of an allyl group attached to the nitrogen atom. This compound has garnered attention for its potential biological activities, including antimicrobial, anticancer, and neuropharmacological effects. This article explores the biological activity of 1-allylpiperidine, presenting findings from various studies and synthesizing relevant data.
1-Allylpiperidine is a colorless liquid at room temperature with a boiling point of approximately 151-152°C. Its structure allows it to interact with various biological systems, primarily targeting the respiratory system. The compound exhibits irritant properties, which can lead to respiratory issues upon exposure. The mechanism of action involves cellular disruption and potential oxidative stress in target tissues, which may contribute to its biological effects.
Antimicrobial Properties
Recent studies have indicated that derivatives of 1-allylpiperidine possess significant antimicrobial activity. For instance, research highlighted the effectiveness of 1-allylpiperidine derivatives against mycobacterial strains, demonstrating both planktonic and dormant cell activity. The following table summarizes findings from various studies on the antimicrobial efficacy of 1-allylpiperidine:
Anticancer Activity
Research has also explored the anticancer properties of 1-allylpiperidine derivatives. A study focused on the synthesis and evaluation of these compounds demonstrated their ability to inhibit cancer cell proliferation in vitro. The compound's mechanism may involve apoptosis induction and cell cycle arrest in cancer cells.
Neuropharmacological Effects
The neuropharmacological profile of 1-allylpiperidine suggests potential applications in treating neurological disorders. Its structural similarity to known psychoactive compounds positions it as a candidate for further investigation in central nervous system (CNS) pharmacology. Studies have indicated that it may act as an NMDA receptor antagonist, which could be beneficial in conditions like Alzheimer's disease or other neurodegenerative disorders.
Case Studies
Case Study 1: Neuroprotective Effects
In a controlled study examining the neuroprotective effects of 1-allylpiperidine in rodent models, researchers observed significant improvements in cognitive function following administration. The study concluded that the compound could mitigate oxidative stress-related neuronal damage.
Case Study 2: Antimicrobial Efficacy
A comparative analysis involving various piperidine derivatives revealed that 1-allylpiperidine exhibited superior antimicrobial activity against resistant strains of bacteria compared to traditional antibiotics. This finding underscores its potential as a novel therapeutic agent in infectious diseases.
Propiedades
IUPAC Name |
1-prop-2-enylpiperidine | |
---|---|---|
Source | PubChem | |
URL | https://pubchem.ncbi.nlm.nih.gov | |
Description | Data deposited in or computed by PubChem | |
InChI |
InChI=1S/C8H15N/c1-2-6-9-7-4-3-5-8-9/h2H,1,3-8H2 | |
Source | PubChem | |
URL | https://pubchem.ncbi.nlm.nih.gov | |
Description | Data deposited in or computed by PubChem | |
InChI Key |
KYGMSGYKSGNPHM-UHFFFAOYSA-N | |
Source | PubChem | |
URL | https://pubchem.ncbi.nlm.nih.gov | |
Description | Data deposited in or computed by PubChem | |
Canonical SMILES |
C=CCN1CCCCC1 | |
Source | PubChem | |
URL | https://pubchem.ncbi.nlm.nih.gov | |
Description | Data deposited in or computed by PubChem | |
Molecular Formula |
C8H15N | |
Source | PubChem | |
URL | https://pubchem.ncbi.nlm.nih.gov | |
Description | Data deposited in or computed by PubChem | |
DSSTOX Substance ID |
DTXSID40162712 | |
Record name | 1-(2-Propenyl)piperidine | |
Source | EPA DSSTox | |
URL | https://comptox.epa.gov/dashboard/DTXSID40162712 | |
Description | DSSTox provides a high quality public chemistry resource for supporting improved predictive toxicology. | |
Molecular Weight |
125.21 g/mol | |
Source | PubChem | |
URL | https://pubchem.ncbi.nlm.nih.gov | |
Description | Data deposited in or computed by PubChem | |
CAS No. |
14446-67-4 | |
Record name | 1-(2-Propen-1-yl)piperidine | |
Source | CAS Common Chemistry | |
URL | https://commonchemistry.cas.org/detail?cas_rn=14446-67-4 | |
Description | CAS Common Chemistry is an open community resource for accessing chemical information. Nearly 500,000 chemical substances from CAS REGISTRY cover areas of community interest, including common and frequently regulated chemicals, and those relevant to high school and undergraduate chemistry classes. This chemical information, curated by our expert scientists, is provided in alignment with our mission as a division of the American Chemical Society. | |
Explanation | The data from CAS Common Chemistry is provided under a CC-BY-NC 4.0 license, unless otherwise stated. | |
Record name | 1-(2-Propenyl)piperidine | |
Source | ChemIDplus | |
URL | https://pubchem.ncbi.nlm.nih.gov/substance/?source=chemidplus&sourceid=0014446674 | |
Description | ChemIDplus is a free, web search system that provides access to the structure and nomenclature authority files used for the identification of chemical substances cited in National Library of Medicine (NLM) databases, including the TOXNET system. | |
Record name | 1-(2-Propenyl)piperidine | |
Source | EPA DSSTox | |
URL | https://comptox.epa.gov/dashboard/DTXSID40162712 | |
Description | DSSTox provides a high quality public chemistry resource for supporting improved predictive toxicology. | |
Synthesis routes and methods I
Procedure details
Synthesis routes and methods II
Procedure details
Synthesis routes and methods III
Procedure details
Synthesis routes and methods IV
Procedure details
Retrosynthesis Analysis
AI-Powered Synthesis Planning: Our tool employs the Template_relevance Pistachio, Template_relevance Bkms_metabolic, Template_relevance Pistachio_ringbreaker, Template_relevance Reaxys, Template_relevance Reaxys_biocatalysis model, leveraging a vast database of chemical reactions to predict feasible synthetic routes.
One-Step Synthesis Focus: Specifically designed for one-step synthesis, it provides concise and direct routes for your target compounds, streamlining the synthesis process.
Accurate Predictions: Utilizing the extensive PISTACHIO, BKMS_METABOLIC, PISTACHIO_RINGBREAKER, REAXYS, REAXYS_BIOCATALYSIS database, our tool offers high-accuracy predictions, reflecting the latest in chemical research and data.
Strategy Settings
Precursor scoring | Relevance Heuristic |
---|---|
Min. plausibility | 0.01 |
Model | Template_relevance |
Template Set | Pistachio/Bkms_metabolic/Pistachio_ringbreaker/Reaxys/Reaxys_biocatalysis |
Top-N result to add to graph | 6 |
Feasible Synthetic Routes
Q1: Can N-Allylpiperidine be used as a building block for synthesizing other complex molecules?
A1: Yes, the provided research highlights N-allylpiperidine's use as a starting material for chemical synthesis. For instance, it can undergo hydrosilylation reactions, a process that attaches silicon-hydrogen bonds across carbon-carbon double bonds. This reaction is useful for creating new silicon-containing compounds with potential applications in various fields [].
Q2: The abstract mentions a "tetrazole-substituted amino acid" with NMDA antagonist activity. Is there a connection between this compound and N-Allylpiperidine?
A2: While not directly stated, the synthesis of the NMDA antagonist (+/-)-(2SR,4RS)-4-(1H-tetrazol-5-ylmethyl)piperidine-2-carboxylic acid (LY233053) likely involves a piperidine derivative as a key intermediate. N-Allylpiperidine itself might not be directly used, but the chemistry involved could share similarities, highlighting the role of piperidine derivatives in medicinal chemistry [].
Q3: The research mentions using N,N-dimethylallylamine and similar compounds. What is the significance of these "allylamines" in relation to N-allylpiperidine?
A3: Both N-allylpiperidine and the mentioned allylamines share a common structural feature: the allyl group (CH2=CH-CH2-). This group is reactive and can participate in various chemical reactions. Studying how different allylamines, including N-allylpiperidine, behave in reactions with boron-containing compounds (like those described in the research) can provide valuable insights into reactivity patterns and guide the development of new synthetic strategies [].
Descargo de responsabilidad e información sobre productos de investigación in vitro
Tenga en cuenta que todos los artículos e información de productos presentados en BenchChem están destinados únicamente con fines informativos. Los productos disponibles para la compra en BenchChem están diseñados específicamente para estudios in vitro, que se realizan fuera de organismos vivos. Los estudios in vitro, derivados del término latino "in vidrio", involucran experimentos realizados en entornos de laboratorio controlados utilizando células o tejidos. Es importante tener en cuenta que estos productos no se clasifican como medicamentos y no han recibido la aprobación de la FDA para la prevención, tratamiento o cura de ninguna condición médica, dolencia o enfermedad. Debemos enfatizar que cualquier forma de introducción corporal de estos productos en humanos o animales está estrictamente prohibida por ley. Es esencial adherirse a estas pautas para garantizar el cumplimiento de los estándares legales y éticos en la investigación y experimentación.