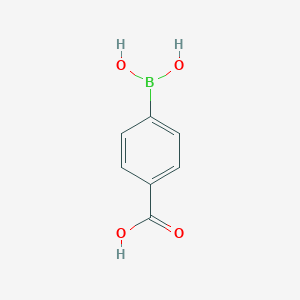
Ácido 4-carboxifenilborónico
Descripción general
Descripción
El Ácido 4-Carboxifenilborónico, también conocido como Ácido 4-Boronobenzoico, es un compuesto orgánico con la fórmula molecular C7H7BO4. Es un derivado del ácido benzoico donde un grupo de ácido borónico está unido al anillo de benceno. Este compuesto se utiliza ampliamente en la síntesis orgánica, particularmente en reacciones de acoplamiento de Suzuki, debido a su capacidad de formar enlaces covalentes estables con dioles y otras moléculas .
Aplicaciones Científicas De Investigación
El Ácido 4-Carboxifenilborónico tiene una amplia gama de aplicaciones en la investigación científica:
Mecanismo De Acción
El mecanismo de acción del Ácido 4-Carboxifenilborónico involucra su capacidad para formar enlaces covalentes estables con dioles y otras moléculas. Esta propiedad se utiliza en varias reacciones, como el acoplamiento de Suzuki, donde forma compuestos biarílicos al acoplarse con haluros en presencia de un catalizador de paladio. El grupo ácido borónico interactúa con los objetivos moleculares, facilitando la formación de nuevos enlaces químicos .
Análisis Bioquímico
Biochemical Properties
4-Carboxyphenylboronic acid is involved in several biochemical reactions, including condensation reactions with stabilizer chains at the surface of polystyrene latex, Suzuki coupling reactions, esterification, derivatization of polyvinylamine, and synthesis of isotopically labeled mercury
Cellular Effects
It has been used in the functionalization of poly-SiNW for the detection of dopamine , suggesting that it may influence cellular processes related to neurotransmitter metabolism
Molecular Mechanism
The molecular mechanism of action of 4-Carboxyphenylboronic acid is largely dependent on the specific biochemical reaction in which it is involved. For instance, in Suzuki-Miyaura cross-coupling reactions, it participates in the formation of carbon-carbon bonds
Metabolic Pathways
The metabolic pathways involving 4-Carboxyphenylboronic acid are not well-defined. It is known to participate in Suzuki-Miyaura cross-coupling reactions
Métodos De Preparación
Rutas Sintéticas y Condiciones de Reacción: La preparación del Ácido 4-Carboxifenilborónico típicamente involucra los siguientes pasos:
Reacción de Esterificación: El ácido yodobenzoico se utiliza como material de partida, que se somete a esterificación para formar un intermedio de éster.
Reacción de Acidificación del Boro: El intermedio de éster luego se somete a acidificación del boro, introduciendo el grupo ácido borónico.
Reacción de Hidrólisis: Finalmente, el éster se hidroliza para producir this compound.
Métodos de Producción Industrial: La producción industrial del this compound sigue rutas sintéticas similares, pero se optimiza para obtener mayores rendimientos y rentabilidad. El proceso involucra el uso de materias primas fácilmente disponibles y pasos simples de postprocesamiento, lo que lo hace adecuado para la producción a gran escala .
Análisis De Reacciones Químicas
El Ácido 4-Carboxifenilborónico experimenta varias reacciones químicas, que incluyen:
Reacciones de Condensación: Reacciona con cadenas estabilizadoras en la superficie del látex de poliestireno.
Reacciones de Acoplamiento de Suzuki: Esta es una reacción clave en la que se acopla con haluros en presencia de un catalizador de paladio para formar compuestos biarílicos.
Esterificación: Puede formar ésteres con alcoholes en condiciones ácidas.
Derivatización de Polivinilamina: Reacciona con polivinilamina para formar derivados.
Oxidación y Reducción: Puede someterse a oxidación para formar fenoles y reducción para formar ésteres de ácido borónico.
Reactivos y Condiciones Comunes:
Catalizadores de Paladio: Utilizados en reacciones de acoplamiento de Suzuki.
Condiciones Ácidas: Necesarias para reacciones de esterificación.
Agentes Oxidantes: Como aire u otros oxidantes para reacciones de oxidación.
Productos Principales:
Compuestos Biarílicos: De reacciones de acoplamiento de Suzuki.
Ésteres: De reacciones de esterificación.
Fenoles: De reacciones de oxidación.
Comparación Con Compuestos Similares
El Ácido 4-Carboxifenilborónico es único debido a sus grupos funcionales carboxilo y ácido borónico, que proporcionan versatilidad en las reacciones químicas. Los compuestos similares incluyen:
Ácido 4-Formilfenilborónico: Se utiliza en reacciones de acoplamiento similares, pero tiene un grupo formilo en lugar de un grupo carboxilo.
Ácido 3-Carboxifenilborónico: Estructura similar, pero con el grupo carboxilo en una posición diferente en el anillo de benceno.
Ácido Fenilborónico: Carece del grupo carboxilo, lo que lo hace menos versátil en ciertas reacciones.
Estos compuestos comparten una reactividad similar, pero difieren en sus grupos funcionales, lo que afecta sus aplicaciones específicas y perfiles de reactividad.
Propiedades
IUPAC Name |
4-boronobenzoic acid | |
---|---|---|
Source | PubChem | |
URL | https://pubchem.ncbi.nlm.nih.gov | |
Description | Data deposited in or computed by PubChem | |
InChI |
InChI=1S/C7H7BO4/c9-7(10)5-1-3-6(4-2-5)8(11)12/h1-4,11-12H,(H,9,10) | |
Source | PubChem | |
URL | https://pubchem.ncbi.nlm.nih.gov | |
Description | Data deposited in or computed by PubChem | |
InChI Key |
SIAVMDKGVRXFAX-UHFFFAOYSA-N | |
Source | PubChem | |
URL | https://pubchem.ncbi.nlm.nih.gov | |
Description | Data deposited in or computed by PubChem | |
Canonical SMILES |
B(C1=CC=C(C=C1)C(=O)O)(O)O | |
Source | PubChem | |
URL | https://pubchem.ncbi.nlm.nih.gov | |
Description | Data deposited in or computed by PubChem | |
Molecular Formula |
C7H7BO4 | |
Record name | 4-carboxyphenylboronic acid | |
Source | Wikipedia | |
URL | https://en.wikipedia.org/wiki/Dictionary_of_chemical_formulas | |
Description | Chemical information link to Wikipedia. | |
Source | PubChem | |
URL | https://pubchem.ncbi.nlm.nih.gov | |
Description | Data deposited in or computed by PubChem | |
DSSTOX Substance ID |
DTXSID20930850 | |
Record name | 4-Boronobenzoic acid | |
Source | EPA DSSTox | |
URL | https://comptox.epa.gov/dashboard/DTXSID20930850 | |
Description | DSSTox provides a high quality public chemistry resource for supporting improved predictive toxicology. | |
Molecular Weight |
165.94 g/mol | |
Source | PubChem | |
URL | https://pubchem.ncbi.nlm.nih.gov | |
Description | Data deposited in or computed by PubChem | |
Physical Description |
Powder; [Alfa Aesar MSDS] | |
Record name | 4-Carboxyphenylboronic acid | |
Source | Haz-Map, Information on Hazardous Chemicals and Occupational Diseases | |
URL | https://haz-map.com/Agents/20011 | |
Description | Haz-Map® is an occupational health database designed for health and safety professionals and for consumers seeking information about the adverse effects of workplace exposures to chemical and biological agents. | |
Explanation | Copyright (c) 2022 Haz-Map(R). All rights reserved. Unless otherwise indicated, all materials from Haz-Map are copyrighted by Haz-Map(R). No part of these materials, either text or image may be used for any purpose other than for personal use. Therefore, reproduction, modification, storage in a retrieval system or retransmission, in any form or by any means, electronic, mechanical or otherwise, for reasons other than personal use, is strictly prohibited without prior written permission. | |
CAS No. |
14047-29-1 | |
Record name | 4-Carboxyphenylboronic acid | |
Source | CAS Common Chemistry | |
URL | https://commonchemistry.cas.org/detail?cas_rn=14047-29-1 | |
Description | CAS Common Chemistry is an open community resource for accessing chemical information. Nearly 500,000 chemical substances from CAS REGISTRY cover areas of community interest, including common and frequently regulated chemicals, and those relevant to high school and undergraduate chemistry classes. This chemical information, curated by our expert scientists, is provided in alignment with our mission as a division of the American Chemical Society. | |
Explanation | The data from CAS Common Chemistry is provided under a CC-BY-NC 4.0 license, unless otherwise stated. | |
Record name | 14047-29-1 | |
Source | DTP/NCI | |
URL | https://dtp.cancer.gov/dtpstandard/servlet/dwindex?searchtype=NSC&outputformat=html&searchlist=221170 | |
Description | The NCI Development Therapeutics Program (DTP) provides services and resources to the academic and private-sector research communities worldwide to facilitate the discovery and development of new cancer therapeutic agents. | |
Explanation | Unless otherwise indicated, all text within NCI products is free of copyright and may be reused without our permission. Credit the National Cancer Institute as the source. | |
Record name | 4-Boronobenzoic acid | |
Source | EPA DSSTox | |
URL | https://comptox.epa.gov/dashboard/DTXSID20930850 | |
Description | DSSTox provides a high quality public chemistry resource for supporting improved predictive toxicology. | |
Record name | 4-CARBOXYPHENYLBORONIC ACID | |
Source | FDA Global Substance Registration System (GSRS) | |
URL | https://gsrs.ncats.nih.gov/ginas/app/beta/substances/XQQ1T11DYK | |
Description | The FDA Global Substance Registration System (GSRS) enables the efficient and accurate exchange of information on what substances are in regulated products. Instead of relying on names, which vary across regulatory domains, countries, and regions, the GSRS knowledge base makes it possible for substances to be defined by standardized, scientific descriptions. | |
Explanation | Unless otherwise noted, the contents of the FDA website (www.fda.gov), both text and graphics, are not copyrighted. They are in the public domain and may be republished, reprinted and otherwise used freely by anyone without the need to obtain permission from FDA. Credit to the U.S. Food and Drug Administration as the source is appreciated but not required. | |
Synthesis routes and methods I
Procedure details
Synthesis routes and methods II
Procedure details
Retrosynthesis Analysis
AI-Powered Synthesis Planning: Our tool employs the Template_relevance Pistachio, Template_relevance Bkms_metabolic, Template_relevance Pistachio_ringbreaker, Template_relevance Reaxys, Template_relevance Reaxys_biocatalysis model, leveraging a vast database of chemical reactions to predict feasible synthetic routes.
One-Step Synthesis Focus: Specifically designed for one-step synthesis, it provides concise and direct routes for your target compounds, streamlining the synthesis process.
Accurate Predictions: Utilizing the extensive PISTACHIO, BKMS_METABOLIC, PISTACHIO_RINGBREAKER, REAXYS, REAXYS_BIOCATALYSIS database, our tool offers high-accuracy predictions, reflecting the latest in chemical research and data.
Strategy Settings
Precursor scoring | Relevance Heuristic |
---|---|
Min. plausibility | 0.01 |
Model | Template_relevance |
Template Set | Pistachio/Bkms_metabolic/Pistachio_ringbreaker/Reaxys/Reaxys_biocatalysis |
Top-N result to add to graph | 6 |
Feasible Synthetic Routes
A: 4-Carboxyphenylboronic acid interacts with its targets primarily through the formation of reversible covalent bonds with 1,3-diol containing molecules. [] This interaction is particularly relevant for recognizing and binding to saccharides, such as glucose, [, , ] due to their abundance in cis-diol groups. This binding event can trigger various downstream effects depending on the specific application. For example, in drug delivery systems, it can lead to the controlled release of insulin in response to varying glucose levels. [, ] In sensing applications, the binding event can generate a detectable signal change, enabling the quantification of specific analytes. [, , , ]
ANone: 4-Carboxyphenylboronic acid features a phenyl ring substituted with both a boronic acid (-B(OH)2) group and a carboxylic acid (-COOH) group at the para positions.
- Spectroscopic Data: Characterization techniques like FTIR, 1H-NMR, and 13C-NMR are frequently employed to confirm the structure and purity of 4-Carboxyphenylboronic acid. [, , , ] These techniques provide information about the presence and environment of specific functional groups within the molecule.
A: 4-Carboxyphenylboronic acid displays compatibility with a wide range of materials, including polymers like poly(vinyl alcohol) (PVA) [, , , ] and chitosan, [] mesoporous silica, [, ] and various nanomaterials like gold nanoclusters [] and carbon dots. [] This versatility enables its application in diverse areas. For instance, it allows the creation of glucose-responsive hydrogels by crosslinking PVA with 4-carboxyphenylboronic acid in the presence of calcium ions. [] Additionally, it facilitates the development of magnetofluorescent nanoparticles for targeted drug delivery and imaging by conjugating with Cdots and MnFe2O4 magnetic nanoparticles. []
ANone: Although specific examples of computational studies on 4-carboxyphenylboronic acid are not extensively discussed in the provided papers, computational chemistry techniques like molecular modeling, docking simulations, and density functional theory calculations could be valuable tools to further investigate the interactions of 4-carboxyphenylboronic acid with its targets, predict binding affinities, and design derivatives with enhanced properties.
A: Structural modifications to 4-carboxyphenylboronic acid can significantly influence its properties and activity. For instance, incorporating fluorine into the phenyl ring, as seen in 3-fluoro-4-carboxyphenylboronic acid, can increase its binding affinity for diols, [] potentially due to the electron-withdrawing nature of fluorine. Furthermore, the position of the boronic acid group on the phenyl ring can impact its interaction with targets. In pseudopolyrotaxanes, the use of 3-carboxy-5-nitrophenylboronic acid, compared to 4-carboxyphenylboronic acid, led to improved glucose sensitivity due to the difference in spatial arrangement and accessibility of the boronic acid group for binding. [] These examples highlight the importance of structure-activity relationship studies for optimizing the desired properties of 4-carboxyphenylboronic acid derivatives.
A: While specific data on the stability of 4-carboxyphenylboronic acid under various conditions is limited in the provided research, it's crucial to consider potential degradation pathways, such as oxidation or hydrolysis, depending on the application. Formulation strategies to enhance stability could involve incorporating antioxidants, adjusting pH, controlling moisture content, and selecting appropriate packaging. For instance, in drug delivery applications, encapsulating 4-carboxyphenylboronic acid within nanoparticles or other delivery vehicles can not only protect it from degradation but also potentially improve its solubility and bioavailability. [, , ]
ANone: Specific SHE regulations concerning 4-carboxyphenylboronic acid may vary depending on geographical location and intended use. It is crucial to consult relevant safety data sheets (SDS) and comply with local regulations for handling, storage, and disposal. Risk assessments should be conducted to identify potential hazards and implement appropriate control measures. As with any chemical, using appropriate personal protective equipment, ensuring proper ventilation, and practicing good laboratory hygiene are essential for minimizing risks and promoting responsible handling.
A: 4-carboxyphenylboronic acid finds applications across multiple disciplines due to its unique properties. It bridges chemistry and material science in the development of novel materials like glucose-responsive hydrogels. [, , , ] Its application in biosensing demonstrates synergy between chemistry, material science, and analytical chemistry. [, , , ] The ability of 4-carboxyphenylboronic acid to facilitate ribonucleoside transport through lipid bilayers highlights its potential in chemical biology and drug delivery research. [] Furthermore, its use in capturing glycoproteins for disease diagnosis and analysis showcases its importance in the biomedical field. [] These examples showcase the interdisciplinary nature of research involving 4-carboxyphenylboronic acid and its potential to drive innovation in various fields.
Descargo de responsabilidad e información sobre productos de investigación in vitro
Tenga en cuenta que todos los artículos e información de productos presentados en BenchChem están destinados únicamente con fines informativos. Los productos disponibles para la compra en BenchChem están diseñados específicamente para estudios in vitro, que se realizan fuera de organismos vivos. Los estudios in vitro, derivados del término latino "in vidrio", involucran experimentos realizados en entornos de laboratorio controlados utilizando células o tejidos. Es importante tener en cuenta que estos productos no se clasifican como medicamentos y no han recibido la aprobación de la FDA para la prevención, tratamiento o cura de ninguna condición médica, dolencia o enfermedad. Debemos enfatizar que cualquier forma de introducción corporal de estos productos en humanos o animales está estrictamente prohibida por ley. Es esencial adherirse a estas pautas para garantizar el cumplimiento de los estándares legales y éticos en la investigación y experimentación.