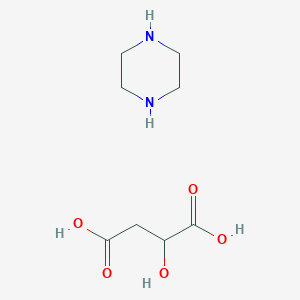
Piperazine DL-Malate
Descripción general
Descripción
Piperazine DL-Malate is a chemical compound with the molecular formula C4H10N2·C4H6O5 and a molecular weight of 220.23. It appears as a white to almost white powder or crystal . This compound is a derivative of piperazine, a heterocyclic organic compound that contains a six-membered ring with two nitrogen atoms at opposite positions. Piperazine derivatives are widely used in pharmaceuticals due to their diverse biological activities.
Mecanismo De Acción
Target of Action
Piperazine DL-Malate, like other piperazine derivatives, primarily targets the GABA receptors . These receptors are a class of receptors that respond to the neurotransmitter gamma-aminobutyric acid (GABA), the chief inhibitory compound in the mature vertebrate central nervous system.
Mode of Action
This compound acts as a GABA receptor agonist . It binds directly and selectively to muscle membrane GABA receptors, presumably causing hyperpolarization of nerve endings . This results in flaccid paralysis of the worm, which is why piperazine compounds are often used as anthelmintic agents .
Biochemical Pathways
It is known that piperazine derivatives can have a significant impact on various biochemical pathways due to their widespread presence in drugs and bioactive molecules
Pharmacokinetics
The piperazine moiety is often used as a basic and hydrophilic group to optimize the pharmacokinetic properties of the final molecule . It is also used as a scaffold to arrange pharmacophoric groups in the proper position in the interaction with the target macromolecules . .
Result of Action
The primary result of this compound’s action is the paralysis of parasites, allowing the host body to easily remove or expel the invading organism . This is why piperazine compounds, including this compound, are often used as anthelmintic agents .
Análisis Bioquímico
Biochemical Properties
The biochemical properties of Piperazine DL-Malate are not fully understood. Piperazine, a component of this compound, is known to interact with various enzymes, proteins, and other biomolecules. It acts as a reversible inhibitor of acetylcholinesterase (AchE), an enzyme that hydrolyzes acetylcholine (Ach) . AchE is involved in the transmission of nervous signals from nerves to muscles and between neurons in the brain. Inhibition of AchE massively disturbs the motility of parasites .
Cellular Effects
Piperazine derivatives have been shown to have cytotoxic effects on cancer cells, inducing apoptosis . These effects are likely due to the disruption of normal cellular signaling pathways, changes in gene expression, and alterations in cellular metabolism .
Molecular Mechanism
The exact molecular mechanism of this compound is not well-known. Piperazine, a component of this compound, is known to exert its effects at the molecular level through various mechanisms. For instance, it acts as a reversible inhibitor of acetylcholinesterase, leading to the disturbance of the motility of parasites .
Temporal Effects in Laboratory Settings
The crystalline salt of this compound has been prepared and characterized by X-ray crystallography , suggesting that it may have stability for use in laboratory settings.
Dosage Effects in Animal Models
While specific dosage effects of this compound in animal models are not documented, piperazine salts are generally well-tolerated by animals. In dogs and cats, single oral doses ≥110 mg/kg can already cause slight adverse drug reactions; ≥800 mg/kg cause neurotoxic symptoms .
Metabolic Pathways
Malate, a component of this compound, is involved in several metabolic pathways, including the tricarboxylic acid (TCA), C4-dicarboxylic acid, and glyoxylate cycles .
Subcellular Localization
Understanding the subcellular localization of a protein or compound is key to understanding its function and mechanism .
Métodos De Preparación
Synthetic Routes and Reaction Conditions: The synthesis of piperazine derivatives, including Piperazine DL-Malate, typically involves the reaction of piperazine with malic acid. The process can be carried out under various conditions, including room temperature or elevated temperatures, and in the presence of solvents such as water or ethanol. The reaction may also involve the use of catalysts to enhance the yield and purity of the product .
Industrial Production Methods: Industrial production of this compound often employs batch or continuous flow reactors. The use of microwave-assisted synthesis has also been explored to improve the efficiency and reduce the reaction time . The final product is usually purified through crystallization or recrystallization techniques to achieve the desired purity levels.
Análisis De Reacciones Químicas
Types of Reactions: Piperazine DL-Malate undergoes various chemical reactions, including:
Oxidation: Piperazine derivatives can be oxidized using oxidizing agents such as meta-chloroperoxybenzoic acid (m-CPBA).
Reduction: Reduction reactions can be carried out using reducing agents like sodium borohydride or lithium aluminum hydride.
Substitution: Nucleophilic substitution reactions are common, where piperazine reacts with alkyl halides or sulfonates to form N-alkylpiperazines.
Common Reagents and Conditions:
Oxidation: m-CPBA in an organic solvent.
Reduction: Sodium borohydride in ethanol or lithium aluminum hydride in ether.
Substitution: Alkyl halides or sulfonates in the presence of a base such as sodium hydroxide.
Major Products:
Oxidation: Formation of N-oxide derivatives.
Reduction: Formation of N-alkylpiperazines.
Substitution: Formation of various substituted piperazine derivatives.
Aplicaciones Científicas De Investigación
Piperazine DL-Malate has a wide range of applications in scientific research, including:
Comparación Con Compuestos Similares
Piperazine: The parent compound, widely used as an anthelmintic agent.
N-alkylpiperazines: Derivatives with various alkyl groups attached to the nitrogen atoms, used in pharmaceuticals.
Piperazine citrate: Another piperazine derivative with similar anthelmintic properties.
Uniqueness of Piperazine DL-Malate: this compound is unique due to its combination with malic acid, which may enhance its solubility and bioavailability.
Actividad Biológica
Piperazine DL-Malate (PDM) is a compound of interest in various scientific fields due to its unique chemical structure and potential biological activities. This article explores the biological activity of PDM, focusing on its mechanisms, applications, and relevant research findings.
Chemical Structure
This compound consists of two main components:
- Piperazine : A six-membered ring containing two nitrogen atoms, known for its role in various biological activities.
- Malic Acid : A dicarboxylic acid that contributes to the compound's solubility and reactivity.
The presence of amine groups in piperazine and carboxylic acid groups in malic acid allows for significant chemical interactions, such as hydrogen bonding and ionic bonding, which can influence its biological activity.
Anthelmintic Effects
Piperazine has been traditionally used as an anthelmintic , particularly effective against intestinal worms. Research indicates that PDM can inhibit the neuromuscular activity of helminths, leading to paralysis and subsequent expulsion from the host .
Immune Modulation
Recent studies suggest that PDM may enhance the identification of mononuclear cells in blood samples from individuals with celiac disease. This property could lead to more sensitive diagnostic tests for autoimmune conditions. The mechanism behind this activity may involve modulation of immune responses through the activation of specific immune pathways.
Study on Celiac Disease
A study highlighted the potential of PDM in increasing the number of identifiable mononuclear cells in patients with celiac disease. This finding suggests a role for PDM in improving diagnostic techniques for autoimmune diseases.
Antiviral Activity in Plants
Research involving novel trifluoromethylpyridine piperazine derivatives demonstrated significant antiviral activity against TMV and Cucumber Mosaic Virus (CMV). These studies indicated that such compounds could induce systemic acquired resistance (SAR) in plants, enhancing their ability to fend off viral infections .
Summary of Biological Activities
Propiedades
IUPAC Name |
2-hydroxybutanedioic acid;piperazine | |
---|---|---|
Source | PubChem | |
URL | https://pubchem.ncbi.nlm.nih.gov | |
Description | Data deposited in or computed by PubChem | |
InChI |
InChI=1S/C4H10N2.C4H6O5/c1-2-6-4-3-5-1;5-2(4(8)9)1-3(6)7/h5-6H,1-4H2;2,5H,1H2,(H,6,7)(H,8,9) | |
Source | PubChem | |
URL | https://pubchem.ncbi.nlm.nih.gov | |
Description | Data deposited in or computed by PubChem | |
InChI Key |
MGQUENZFJVXCFB-UHFFFAOYSA-N | |
Source | PubChem | |
URL | https://pubchem.ncbi.nlm.nih.gov | |
Description | Data deposited in or computed by PubChem | |
Canonical SMILES |
C1CNCCN1.C(C(C(=O)O)O)C(=O)O | |
Source | PubChem | |
URL | https://pubchem.ncbi.nlm.nih.gov | |
Description | Data deposited in or computed by PubChem | |
Molecular Formula |
C8H16N2O5 | |
Source | PubChem | |
URL | https://pubchem.ncbi.nlm.nih.gov | |
Description | Data deposited in or computed by PubChem | |
DSSTOX Substance ID |
DTXSID50608257 | |
Record name | 2-Hydroxybutanedioic acid--piperazine (1/1) | |
Source | EPA DSSTox | |
URL | https://comptox.epa.gov/dashboard/DTXSID50608257 | |
Description | DSSTox provides a high quality public chemistry resource for supporting improved predictive toxicology. | |
Molecular Weight |
220.22 g/mol | |
Source | PubChem | |
URL | https://pubchem.ncbi.nlm.nih.gov | |
Description | Data deposited in or computed by PubChem | |
CAS No. |
14852-14-3 | |
Record name | 2-Hydroxybutanedioic acid--piperazine (1/1) | |
Source | EPA DSSTox | |
URL | https://comptox.epa.gov/dashboard/DTXSID50608257 | |
Description | DSSTox provides a high quality public chemistry resource for supporting improved predictive toxicology. | |
Descargo de responsabilidad e información sobre productos de investigación in vitro
Tenga en cuenta que todos los artículos e información de productos presentados en BenchChem están destinados únicamente con fines informativos. Los productos disponibles para la compra en BenchChem están diseñados específicamente para estudios in vitro, que se realizan fuera de organismos vivos. Los estudios in vitro, derivados del término latino "in vidrio", involucran experimentos realizados en entornos de laboratorio controlados utilizando células o tejidos. Es importante tener en cuenta que estos productos no se clasifican como medicamentos y no han recibido la aprobación de la FDA para la prevención, tratamiento o cura de ninguna condición médica, dolencia o enfermedad. Debemos enfatizar que cualquier forma de introducción corporal de estos productos en humanos o animales está estrictamente prohibida por ley. Es esencial adherirse a estas pautas para garantizar el cumplimiento de los estándares legales y éticos en la investigación y experimentación.