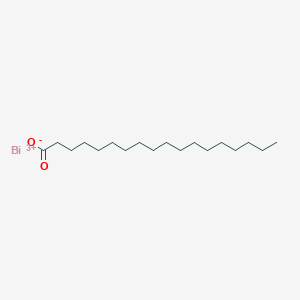
Bismuth(3+) stearate
- Haga clic en CONSULTA RÁPIDA para recibir una cotización de nuestro equipo de expertos.
- Con productos de calidad a un precio COMPETITIVO, puede centrarse más en su investigación.
Descripción general
Descripción
Bismuth(3+) stearate, also known as bismuth tris(stearate), is a coordination compound formed between bismuth ions and stearic acid. It is a white or off-white powder that is insoluble in water but soluble in organic solvents. This compound is often used in various industrial and pharmaceutical applications due to its unique properties, such as its ability to act as a lubricant, stabilizer, and antimicrobial agent .
Métodos De Preparación
Synthetic Routes and Reaction Conditions: Bismuth(3+) stearate can be synthesized through the reaction of bismuth nitrate with sodium stearate in an aqueous medium. The reaction typically occurs at elevated temperatures (around 80°C) and a controlled pH of approximately 1. The resulting product is then precipitated, filtered, and dried .
Industrial Production Methods: In industrial settings, the production of this compound often involves the use of large-scale reactors where bismuth nitrate and sodium stearate are mixed under controlled conditions. The reaction mixture is maintained at a specific temperature and pH to ensure complete reaction and high yield. The precipitated this compound is then collected, washed, and dried to obtain the final product .
Análisis De Reacciones Químicas
Types of Reactions: Bismuth(3+) stearate undergoes various chemical reactions, including thermal decomposition, oxidation, and complexation.
Common Reagents and Conditions:
Thermal Decomposition: When heated, this compound decomposes to form bismuth oxide and stearic acid.
Complexation: this compound can form complexes with various ligands, such as phosphines and amines, under appropriate conditions.
Major Products Formed:
Aplicaciones Científicas De Investigación
Bismuth(3+) stearate has a wide range of applications in scientific research, including:
Mecanismo De Acción
The mechanism of action of bismuth(3+) stearate involves its ability to interact with biological membranes and proteins. Bismuth ions can bind to thiol groups in proteins, leading to the disruption of protein function and microbial cell death. Additionally, the stearate component can integrate into lipid membranes, altering membrane fluidity and permeability .
Comparación Con Compuestos Similares
Bismuth(III) oxide: A common bismuth compound used in various applications, including catalysis and materials science.
Bismuth(III) chloride: Used in organic synthesis and as a reagent in chemical reactions.
Bismuth(III) nitrate: A precursor for the synthesis of other bismuth compounds and used in analytical chemistry.
Uniqueness of Bismuth(3+) Stearate: this compound is unique due to its combination of bismuth’s antimicrobial properties and stearic acid’s ability to act as a lubricant and stabilizer. This dual functionality makes it particularly useful in applications where both antimicrobial activity and lubrication are required .
Actividad Biológica
Bismuth(3+) stearate is a bismuth-based compound that has garnered attention for its potential biological activities, particularly in the treatment of gastrointestinal ailments and as an antimicrobial agent. This article delves into its biological activity, mechanisms of action, and relevant case studies, supported by data tables and research findings.
Overview of Bismuth Compounds
Bismuth compounds, including this compound, are primarily known for their applications in treating ulcers caused by Helicobacter pylori and other gastrointestinal disorders. Bismuth exhibits low bioavailability, with less than 1% of orally ingested bismuth being absorbed into the bloodstream, which limits its systemic toxicity but also its therapeutic efficacy .
This compound acts through several mechanisms:
- Formation of Protective Coatings : It creates a protective barrier over ulcerated tissues, preventing erosion from gastric acid and promoting healing.
- Antimicrobial Activity : Bismuth compounds can disrupt bacterial metabolism by inhibiting critical enzymes such as urease and F1-ATPase, which are essential for H. pylori survival .
- Oxidative Stress Induction : Bismuth has been shown to induce oxidative stress in bacteria, leading to cellular damage and death. It interacts with various proteins involved in oxidative defense systems, effectively impairing bacterial growth .
- Multi-Targeted Approach : Recent studies have indicated that bismuth compounds may target multiple pathways in H. pylori, including the downregulation of virulence factors like CagA and VacA, and disruption of flagella assembly .
Case Studies
-
Efficacy Against H. pylori :
- A study demonstrated that a binuclear Bi(3+) complex exhibited a minimum inhibitory concentration (MIC) of 8.84 µM against H. pylori, significantly more effective than traditional bismuth compounds .
- Another research highlighted that bismuth compounds could inhibit the growth of six different H. pylori strains through integrative proteomic and metabolomic analyses, showcasing their potential in overcoming antibiotic resistance .
- Antimicrobial Properties :
Data Table: Biological Activity Summary
Compound | Target Pathogen | MIC (µM) | Mechanism of Action |
---|---|---|---|
This compound | H. pylori | 8.84 | Inhibition of urease and F1-ATPase |
Binuclear Bi(3+) | H. pylori | 8.84 | Disruption of metabolic pathways |
Bi(3+) complex | MRSA | 0.63-1.25 | Antimicrobial activity against resistant strains |
Bi(3+) coordination polymer | VRE | 0.63-1.25 | Multi-targeted inhibition |
Propiedades
IUPAC Name |
bismuth;octadecanoate |
Source
|
---|---|---|
Source | PubChem | |
URL | https://pubchem.ncbi.nlm.nih.gov | |
Description | Data deposited in or computed by PubChem | |
InChI |
InChI=1S/C18H36O2.Bi/c1-2-3-4-5-6-7-8-9-10-11-12-13-14-15-16-17-18(19)20;/h2-17H2,1H3,(H,19,20);/q;+3/p-1 |
Source
|
Source | PubChem | |
URL | https://pubchem.ncbi.nlm.nih.gov | |
Description | Data deposited in or computed by PubChem | |
InChI Key |
GPHHBRQMPXNXEB-UHFFFAOYSA-M |
Source
|
Source | PubChem | |
URL | https://pubchem.ncbi.nlm.nih.gov | |
Description | Data deposited in or computed by PubChem | |
Canonical SMILES |
CCCCCCCCCCCCCCCCCC(=O)[O-].[Bi+3] |
Source
|
Source | PubChem | |
URL | https://pubchem.ncbi.nlm.nih.gov | |
Description | Data deposited in or computed by PubChem | |
Molecular Formula |
C18H35BiO2+2 |
Source
|
Source | PubChem | |
URL | https://pubchem.ncbi.nlm.nih.gov | |
Description | Data deposited in or computed by PubChem | |
Molecular Weight |
492.4 g/mol |
Source
|
Source | PubChem | |
URL | https://pubchem.ncbi.nlm.nih.gov | |
Description | Data deposited in or computed by PubChem | |
CAS No. |
13283-68-6 |
Source
|
Record name | Bismuth(3+) stearate | |
Source | ChemIDplus | |
URL | https://pubchem.ncbi.nlm.nih.gov/substance/?source=chemidplus&sourceid=0013283686 | |
Description | ChemIDplus is a free, web search system that provides access to the structure and nomenclature authority files used for the identification of chemical substances cited in National Library of Medicine (NLM) databases, including the TOXNET system. | |
Record name | Bismuth(3+) stearate | |
Source | European Chemicals Agency (ECHA) | |
URL | https://echa.europa.eu/substance-information/-/substanceinfo/100.032.982 | |
Description | The European Chemicals Agency (ECHA) is an agency of the European Union which is the driving force among regulatory authorities in implementing the EU's groundbreaking chemicals legislation for the benefit of human health and the environment as well as for innovation and competitiveness. | |
Explanation | Use of the information, documents and data from the ECHA website is subject to the terms and conditions of this Legal Notice, and subject to other binding limitations provided for under applicable law, the information, documents and data made available on the ECHA website may be reproduced, distributed and/or used, totally or in part, for non-commercial purposes provided that ECHA is acknowledged as the source: "Source: European Chemicals Agency, http://echa.europa.eu/". Such acknowledgement must be included in each copy of the material. ECHA permits and encourages organisations and individuals to create links to the ECHA website under the following cumulative conditions: Links can only be made to webpages that provide a link to the Legal Notice page. | |
Descargo de responsabilidad e información sobre productos de investigación in vitro
Tenga en cuenta que todos los artículos e información de productos presentados en BenchChem están destinados únicamente con fines informativos. Los productos disponibles para la compra en BenchChem están diseñados específicamente para estudios in vitro, que se realizan fuera de organismos vivos. Los estudios in vitro, derivados del término latino "in vidrio", involucran experimentos realizados en entornos de laboratorio controlados utilizando células o tejidos. Es importante tener en cuenta que estos productos no se clasifican como medicamentos y no han recibido la aprobación de la FDA para la prevención, tratamiento o cura de ninguna condición médica, dolencia o enfermedad. Debemos enfatizar que cualquier forma de introducción corporal de estos productos en humanos o animales está estrictamente prohibida por ley. Es esencial adherirse a estas pautas para garantizar el cumplimiento de los estándares legales y éticos en la investigación y experimentación.