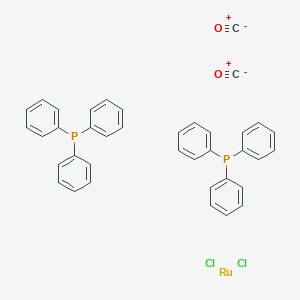
Ruthenium, dicarbonyldichlorobis(triphenylphosphine)-
Descripción general
Descripción
Ruthenium, dicarbonyldichlorobis(triphenylphosphine)- (CAS 14564-35-3) is a ruthenium(II) complex with the molecular formula C₃₈H₃₀Cl₂O₂P₂Ru and a molecular weight of 752.5671 g/mol. It features an octahedral geometry with two carbonyl (CO) ligands, two chloride (Cl⁻) ligands, and two triphenylphosphine (PPh₃) ligands . The CO ligands act as strong π-acceptors, stabilizing the ruthenium center and influencing its electronic properties. This complex is notable for its stability and applications in catalysis, particularly in reactions requiring inert ligand environments .
Métodos De Preparación
Synthetic Routes and Reaction Conditions
The synthesis of Ruthenium, dicarbonyldichlorobis(triphenylphosphine)- typically involves the reaction of ruthenium trichloride hydrate with triphenylphosphine and carbon monoxide. The reaction is carried out in an organic solvent, such as tetrahydrofuran, under an inert atmosphere to prevent oxidation. The mixture is heated to facilitate the formation of the complex .
Industrial Production Methods
In an industrial setting, the preparation of this compound can be scaled up by using larger reaction vessels and maintaining strict control over reaction conditions, such as temperature and pressure, to ensure high yield and purity. The use of automated systems for the addition of reagents and monitoring of reaction progress can further enhance efficiency and safety .
Análisis De Reacciones Químicas
Redox and Hydride Formation
The complex participates in hydrogenation and hydride transfer reactions. In the presence of hydrogen and a base (e.g., NEt₃), it forms chlorohydridotris(triphenylphosphine)ruthenium(II) :
textRuCl₂(CO)₂(PPh₃)₂ + H₂ + NEt₃ → HRuCl(CO)(PPh₃)₃ + [HNEt₃]Cl
This hydride intermediate serves as a catalyst for alkene hydrogenation and transfer hydrogenation of ketones .
Table 2: Redox Reactions
Substrate | Conditions | Product | Application | Reference |
---|---|---|---|---|
H₂ + NEt₃ | Benzene, 25°C | HRuCl(CO)(PPh₃)₃ | Hydrogenation catalysis | |
tert-butyl hydroperoxide | Acetone, reflux | Ru-oxo intermediates | Oxidation of alcohols |
Hydrosilylation
RuCl₂(CO)₂(PPh₃)₂ catalyzes the hydrosilylation of alkenes with silanes. A DFT study revealed a mechanism involving:
-
Oxidative addition of Si–H to Ru(II).
-
Alkene insertion into the Ru–H bond.
Table 3: Hydrosilylation of Methylvinyldimethoxysilane
Catalyst Loading | Silane | Yield (%) | Selectivity (anti-Markovnikov) | Reference |
---|---|---|---|---|
1 mol% | MeSiH(OCH₂CH₃)₂ | 92 | >95% |
Cross-Coupling and C–H Activation
The complex enables C–C bond formation via alcohol cross-coupling through sp³ C–H activation. For example, it catalyzes the alkylation of amines with alcohols via a "borrowing hydrogen" mechanism .
Oxidative Reactions
RuCl₂(CO)₂(PPh₃)₂ oxidizes alkanes to tertiary alcohols and alcohols to ketones using tert-butyl hydroperoxide (TBHP) or O₂. For example:
textR–CH₂–OH → R–C=O (TBHP, acetone, 60°C)
The reaction proceeds via Ru–oxo intermediates detected via in situ spectroscopy .
Reactivity with Lewis Acids
In the presence of Lewis acids (e.g., AlCl₃), RuCl₂(CO)₂(PPh₃)₂ activates C–H bonds in alkanes, enabling functionalization at tertiary positions .
Photochemical Behavior
Under UV irradiation, the complex undergoes ligand dissociation, forming reactive intermediates for small-molecule activation (e.g., O₂, H₂). For example:
textRuCl₂(CO)₂(PPh₃)₂ + hν → RuCl₂(CO)(PPh₃)₂ + CO
The photolyzed species oxygenates in air to form peroxo complexes .
Aplicaciones Científicas De Investigación
Catalytic Applications
1. Hydrogenation Reactions
Ruthenium, dicarbonyldichlorobis(triphenylphosphine) serves as an effective catalyst in hydrogenation reactions. It facilitates the conversion of alkenes, ketones, and nitro compounds into their corresponding saturated products. The catalyst's efficiency stems from its ability to activate hydrogen gas under mild conditions.
Case Study: Hydrogenation of Alkenes
- Reaction:
- Outcome: High yields of saturated hydrocarbons were achieved with minimal side reactions, demonstrating the catalyst's selectivity and efficiency.
2. C-H Activation and Functionalization
The compound has been utilized in C-H activation processes, allowing for the functionalization of hydrocarbons. This method is particularly valuable in organic synthesis as it enables the transformation of inert C-H bonds into more reactive sites.
Case Study: C-H Activation of Aromatics
- Reaction:
- Outcome: The reaction successfully introduced functional groups at specific positions on aromatic rings, showcasing the compound's utility in synthetic organic chemistry.
Oxidative Reactions
1. Oxidation of Alcohols
Ruthenium, dicarbonyldichlorobis(triphenylphosphine) is effective in oxidizing alcohols to aldehydes or ketones using various oxidants such as tert-butyl hydroperoxide.
Case Study: Alcohol Oxidation
- Reaction:
- Outcome: The oxidation process yielded high conversions with good selectivity for the desired carbonyl products.
Cross-Coupling Reactions
The compound plays a crucial role as a catalyst in cross-coupling reactions, particularly in forming carbon-carbon bonds through C-H activation mechanisms.
Case Study: Suzuki Coupling Reaction
- Reaction:
- Outcome: The reaction demonstrated excellent yields and selectivity for biphenyl compounds, highlighting its significance in pharmaceutical synthesis.
Summary Table of Applications
Application Type | Reaction Type | Key Products | Efficiency |
---|---|---|---|
Hydrogenation | Alkenes to Alkanes | Saturated hydrocarbons | High |
C-H Activation | Functionalization of Aromatics | Functionalized aromatics | Moderate |
Oxidation | Alcohols to Aldehydes/Ketones | Aldehydes/Ketones | High |
Cross-Coupling | Suzuki Coupling | Biphenyl compounds | Excellent |
Mecanismo De Acción
The mechanism by which Ruthenium, dicarbonyldichlorobis(triphenylphosphine)- exerts its effects involves the coordination of the ruthenium center with various ligands. This coordination facilitates the activation of substrates, allowing for efficient catalysis of chemical reactions. The molecular targets and pathways involved depend on the specific reaction being catalyzed, but generally involve the formation of intermediate complexes that lower the activation energy of the reaction .
Comparación Con Compuestos Similares
Comparison with Similar Ruthenium Complexes
Dichlorotris(triphenylphosphine)ruthenium(II) [RuCl₂(PPh₃)₃]
- Structure : Contains three PPh₃ ligands and two Cl⁻ ligands.
- Electronic Properties : The additional PPh₃ ligand increases electron density at the Ru center compared to the dicarbonyl complex, making it more nucleophilic .
- Reactivity : Undergoes ligand substitution reactions with amines, nitriles, and β-diketones to form derivatives like RuCl₂(amine)₂(PPh₃)₂ and Ru(β-diket)₂(PPh₃)₂ .
- Bond Lengths : Ru–Cl bond length is 2.387(7) Å , shorter than in the dicarbonyl complex (2.453 Å), indicating stronger Ru–Cl interactions in the absence of CO ligands .
Tetrakis(triphenylphosphine)dichlororuthenium(II) [RuCl₂(PPh₃)₄]
- Structure : Four PPh₃ ligands and two Cl⁻ ligands.
- Catalytic Activity : Used in hydrogenation reactions due to its electron-rich Ru center, which facilitates substrate binding .
- Stability : Less stable than the dicarbonyl complex under oxidative conditions due to weaker π-acceptor ligands .
Chlorocyclopentadienylbis(triphenylphosphine)ruthenium(II) [RuCl(Cp)(PPh₃)₂]
- Structure : Features a cyclopentadienyl (Cp) ligand replacing one CO ligand.
- Electronic Effects : The Cp ligand provides strong σ-donation, enhancing the Ru center’s electron density. This complex exhibits higher activity in C–H bond activation compared to the dicarbonyl analogue .
Dichlorobis(triphenylphosphine)palladium(II) [PdCl₂(PPh₃)₂]
- Cross-Metal Comparison : While structurally similar (two PPh₃ and two Cl⁻ ligands), the Pd(II) complex is less redox-active than Ru(II) complexes. It is widely used in cross-coupling reactions (e.g., Suzuki-Miyaura) but lacks catalytic versatility in CO-involving reactions .
Key Comparative Data
Actividad Biológica
Ruthenium complexes have garnered significant interest in the field of medicinal chemistry, particularly for their potential as anticancer agents. Among these, Ruthenium, dicarbonyldichlorobis(triphenylphosphine) (often referred to as Ru(CO)₂Cl₂(PPh₃)₂) stands out due to its unique biological properties and mechanisms of action. This article reviews the biological activity of this compound, focusing on its cytotoxicity, mechanisms of action, and potential therapeutic applications.
1. Synthesis and Characterization
Ruthenium complexes can be synthesized through various methods, including refluxing dichlorotris(triphenylphosphine)ruthenium(II) with ligands such as bipyridyl or phenanthroline. The resulting compounds exhibit distinct structural and electronic properties that influence their biological activity .
Table 1: Synthesis Methods for Ruthenium Complexes
2.1 Cytotoxicity
Numerous studies have demonstrated the cytotoxic effects of Ru(CO)₂Cl₂(PPh₃)₂ against various cancer cell lines. For instance, it has shown significant activity against triple-negative breast cancer (TNBC) and estrogen receptor-positive breast cancer cells.
- IC₅₀ Values : The IC₅₀ values for Ru(CO)₂Cl₂(PPh₃)₂ in different cancer cell lines are promising:
The mechanisms through which Ru(CO)₂Cl₂(PPh₃)₂ exerts its cytotoxic effects include:
- DNA Interaction : The compound interferes with DNA repair mechanisms and induces apoptosis in cancer cells.
- Mitochondrial Targeting : It has been observed to interact with mitochondrial membranes, leading to increased reactive oxygen species (ROS) generation, which contributes to cell death.
- Cytoskeletal Disruption : The complex affects the cytoskeleton dynamics, inhibiting cell proliferation and colony formation .
3.1 In Vitro Studies
A study by Moreira et al. highlighted the effectiveness of Ru(CO)₂Cl₂(PPh₃)₂ in inducing apoptosis in breast cancer cell lines through mitochondrial pathways. The compound demonstrated a dose-dependent increase in cytotoxicity, particularly in resistant cancer cells .
3.2 In Vivo Studies
In vivo studies using zebrafish models indicated that Ru(CO)₂Cl₂(PPh₃)₂ exhibited low toxicity while maintaining significant anticancer activity. This suggests a favorable therapeutic window for further development as a potential chemotherapeutic agent .
Q & A
Basic Research Questions
Q. How can researchers design a synthesis protocol for ruthenium dicarbonyldichlorobis(triphenylphosphine) while minimizing ligand substitution or decomposition?
- Methodological Answer : Use inert atmosphere techniques (e.g., Schlenk line) to prevent oxidation or hydrolysis. Monitor reaction progress via IR spectroscopy to track CO ligand vibrations (ν(CO) ~1900–2100 cm⁻¹) and confirm retention of the carbonyl groups. Purification via recrystallization in non-polar solvents (e.g., dichloromethane/hexane) can isolate the target complex while avoiding phosphine ligand dissociation .
Q. What spectroscopic techniques are critical for confirming the octahedral geometry and ligand coordination in this complex?
- Methodological Answer :
- X-ray crystallography : Resolve the spatial arrangement of ligands and bond lengths (e.g., Ru–P distances ~2.3–2.4 Å, Ru–Cl ~2.4–2.5 Å) to confirm distorted octahedral geometry .
- NMR spectroscopy : Analyze ³¹P NMR to verify triphenylphosphine coordination (δ ~30–40 ppm for Ru-bound PPh₃) and distinguish free ligands .
- IR spectroscopy : Detect CO stretching frequencies to assess electronic effects of the phosphine ligands .
Q. How does the electronic nature of triphenylphosphine ligands influence the catalytic activity of this complex in CO-related reactions?
- Methodological Answer : Compare turnover frequencies (TOF) in model reactions (e.g., hydroformylation) using complexes with varying phosphine ligands (e.g., electron-rich vs. electron-poor). Use cyclic voltammetry to measure redox potentials (Ru²⁺/Ru³⁺) and correlate with ligand donor strength. DFT calculations can map ligand-induced electron density changes at the Ru center .
Advanced Research Questions
Q. How can DFT studies resolve contradictions in proposed substitution mechanisms for chloride ligands in this complex?
- Methodological Answer : Perform geometry optimization and transition-state analysis using hybrid functionals (e.g., B3LYP) with LanL2DZ basis sets for Ru. Compare activation energies for associative vs. dissociative pathways. Validate computational models against experimental kinetics (e.g., Eyring plots) and isotopic labeling studies to track ligand exchange .
Q. What experimental strategies can differentiate between σ-donor and π-acceptor contributions of CO ligands in stabilizing the Ru center?
- Methodological Answer :
- Resonance Raman spectroscopy : Probe CO vibrational modes under varying excitation wavelengths to isolate π-backbonding effects.
- X-ray absorption spectroscopy (XAS) : Analyze Ru L-edge spectra to quantify d-orbital splitting and ligand-field strength.
- Substitution reactions : Replace CO with stronger π-acceptors (e.g., NO⁺) and monitor changes in redox behavior .
Q. How can researchers reconcile discrepancies in crystallographic data and solution-state NMR observations for this complex?
- Methodological Answer : Perform variable-temperature NMR to detect dynamic processes (e.g., ligand fluxionality) that may mask static crystallographic structures. Use small-angle X-ray scattering (SAXS) in solution to compare with solid-state geometries. Theoretical MD simulations can model solvent effects on ligand conformations .
Q. What mechanistic insights can be gained by studying the anticancer activity of this complex compared to platinum-based drugs?
- Methodological Answer : Conduct comparative DNA binding assays (e.g., ethidium displacement, gel electrophoresis) to assess intercalation or covalent adduct formation. Use ICP-MS to quantify cellular Ru uptake and localization. Pair with transcriptomic profiling to identify Ru-specific apoptotic pathways distinct from cisplatin .
Q. Methodological Challenges and Solutions
Q. How can researchers detect and quantify phosphine ligand dissociation byproducts during catalytic cycles?
- Methodological Answer : Employ ³¹P NMR with ¹H decoupling to identify free PPh₃ (δ ~−5 ppm). Use mass spectrometry (ESI-MS) to track ligand loss in real-time. Introduce chelating agents (e.g., EDTA) to sequester dissociated Ru species and inhibit recombination .
Q. What strategies optimize the stability of this complex under aerobic conditions for prolonged catalytic applications?
- Methodological Answer :
- Ligand modification : Introduce bulky substituents on PPh₃ (e.g., tri-ortho-tolylphosphine) to sterically shield the Ru center.
- Additives : Include sacrificial reductants (e.g., ascorbic acid) to mitigate oxidation.
- Encapsulation : Embed the complex in mesoporous silica or metal-organic frameworks (MOFs) to restrict O₂ access .
Q. How do solvent polarity and coordination strength impact the catalytic selectivity of this complex in asymmetric reactions?
- Methodological Answer : Screen solvents with varying donor numbers (e.g., THF vs. DMF) to modulate Ru–ligand lability. Use chiral additives (e.g., BINAP) to induce enantioselectivity. Monitor enantiomeric excess (ee) via chiral HPLC and correlate with solvent dielectric constants .
Propiedades
IUPAC Name |
carbon monoxide;ruthenium(2+);triphenylphosphane;dichloride | |
---|---|---|
Details | Computed by Lexichem TK 2.7.0 (PubChem release 2021.05.07) | |
Source | PubChem | |
URL | https://pubchem.ncbi.nlm.nih.gov | |
Description | Data deposited in or computed by PubChem | |
InChI |
InChI=1S/2C18H15P.2CO.2ClH.Ru/c2*1-4-10-16(11-5-1)19(17-12-6-2-7-13-17)18-14-8-3-9-15-18;2*1-2;;;/h2*1-15H;;;2*1H;/q;;;;;;+2/p-2 | |
Details | Computed by InChI 1.0.6 (PubChem release 2021.05.07) | |
Source | PubChem | |
URL | https://pubchem.ncbi.nlm.nih.gov | |
Description | Data deposited in or computed by PubChem | |
InChI Key |
PXURRFCLQDNZOY-UHFFFAOYSA-L | |
Details | Computed by InChI 1.0.6 (PubChem release 2021.05.07) | |
Source | PubChem | |
URL | https://pubchem.ncbi.nlm.nih.gov | |
Description | Data deposited in or computed by PubChem | |
Canonical SMILES |
[C-]#[O+].[C-]#[O+].C1=CC=C(C=C1)P(C2=CC=CC=C2)C3=CC=CC=C3.C1=CC=C(C=C1)P(C2=CC=CC=C2)C3=CC=CC=C3.[Cl-].[Cl-].[Ru+2] | |
Details | Computed by OEChem 2.3.0 (PubChem release 2021.05.07) | |
Source | PubChem | |
URL | https://pubchem.ncbi.nlm.nih.gov | |
Description | Data deposited in or computed by PubChem | |
Molecular Formula |
C38H30Cl2O2P2Ru | |
Details | Computed by PubChem 2.1 (PubChem release 2021.05.07) | |
Source | PubChem | |
URL | https://pubchem.ncbi.nlm.nih.gov | |
Description | Data deposited in or computed by PubChem | |
Molecular Weight |
752.6 g/mol | |
Details | Computed by PubChem 2.1 (PubChem release 2021.05.07) | |
Source | PubChem | |
URL | https://pubchem.ncbi.nlm.nih.gov | |
Description | Data deposited in or computed by PubChem | |
CAS No. |
14564-35-3 | |
Record name | Dicarbonyldichlorobis(triphenylphosphine)ruthenium | |
Source | CAS Common Chemistry | |
URL | https://commonchemistry.cas.org/detail?cas_rn=14564-35-3 | |
Description | CAS Common Chemistry is an open community resource for accessing chemical information. Nearly 500,000 chemical substances from CAS REGISTRY cover areas of community interest, including common and frequently regulated chemicals, and those relevant to high school and undergraduate chemistry classes. This chemical information, curated by our expert scientists, is provided in alignment with our mission as a division of the American Chemical Society. | |
Explanation | The data from CAS Common Chemistry is provided under a CC-BY-NC 4.0 license, unless otherwise stated. | |
Descargo de responsabilidad e información sobre productos de investigación in vitro
Tenga en cuenta que todos los artículos e información de productos presentados en BenchChem están destinados únicamente con fines informativos. Los productos disponibles para la compra en BenchChem están diseñados específicamente para estudios in vitro, que se realizan fuera de organismos vivos. Los estudios in vitro, derivados del término latino "in vidrio", involucran experimentos realizados en entornos de laboratorio controlados utilizando células o tejidos. Es importante tener en cuenta que estos productos no se clasifican como medicamentos y no han recibido la aprobación de la FDA para la prevención, tratamiento o cura de ninguna condición médica, dolencia o enfermedad. Debemos enfatizar que cualquier forma de introducción corporal de estos productos en humanos o animales está estrictamente prohibida por ley. Es esencial adherirse a estas pautas para garantizar el cumplimiento de los estándares legales y éticos en la investigación y experimentación.