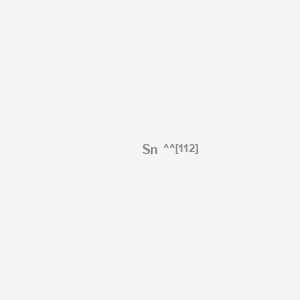
Tin-112
- Haga clic en CONSULTA RÁPIDA para recibir una cotización de nuestro equipo de expertos.
- Con productos de calidad a un precio COMPETITIVO, puede centrarse más en su investigación.
Descripción general
Descripción
Tin-112, is a stable isotope of tin with an atomic number of 50 and a mass number of 112. It contains 50 protons and 62 neutrons in its nucleus. This isotope is one of the ten stable isotopes of tin, which is a post-transition metal known for its malleability and resistance to corrosion .
Métodos De Preparación
Synthetic Routes and Reaction Conditions: Tin-112, can be produced through various nuclear reactions. One common method involves the irradiation of antimony or indium targets with neutrons, which results in the formation of tin isotopes, including tin, isotope of mass 112 .
Industrial Production Methods: In industrial settings, tin isotopes are often separated and enriched using techniques such as gas centrifugation or electromagnetic separation. These methods allow for the production of tin, isotope of mass 112, with high purity and specific enrichment levels .
Análisis De Reacciones Químicas
Types of Reactions: Tin-112, can undergo various chemical reactions, including oxidation, reduction, and substitution reactions.
Common Reagents and Conditions:
Oxidation: this compound, can be oxidized by strong oxidizing agents such as nitric acid, resulting in the formation of tin dioxide.
Reduction: It can be reduced by reducing agents like hydrogen gas or carbon monoxide, leading to the formation of elemental tin.
Substitution: this compound, can participate in substitution reactions with halogens, forming compounds such as tin(IV) chloride.
Major Products Formed:
Oxidation: Tin dioxide
Reduction: Elemental tin
Substitution: Tin(IV) chloride
Aplicaciones Científicas De Investigación
Tin-112, has a wide range of applications in scientific research:
Chemistry: It is used as a tracer in chemical reactions to study reaction mechanisms and pathways.
Biology: Tin isotopes are used in biological labeling to track the movement and distribution of tin in biological systems.
Medicine: this compound, is used in the production of radiopharmaceuticals for diagnostic imaging and therapeutic purposes.
Industry: It is used in the development of advanced materials and in the study of tin-based alloys
Mecanismo De Acción
Tin-112, can be compared with other stable isotopes of tin, such as tin, isotope of mass 114, and tin, isotope of mass 120. While all these isotopes share similar chemical properties, their unique neutron numbers result in different nuclear properties, such as binding energy and separation energy. This makes each isotope suitable for specific applications. For example, tin, isotope of mass 120, is more abundant and commonly used in industrial applications, whereas tin, isotope of mass 112, is preferred for specialized scientific research due to its specific nuclear properties .
Comparación Con Compuestos Similares
- Tin, isotope of mass 114
- Tin, isotope of mass 115
- Tin, isotope of mass 116
- Tin, isotope of mass 117
- Tin, isotope of mass 118
- Tin, isotope of mass 119
- Tin, isotope of mass 120
- Tin, isotope of mass 122
- Tin, isotope of mass 124
Propiedades
IUPAC Name |
tin-112 |
Source
|
---|---|---|
Source | PubChem | |
URL | https://pubchem.ncbi.nlm.nih.gov | |
Description | Data deposited in or computed by PubChem | |
InChI |
InChI=1S/Sn/i1-7 |
Source
|
Source | PubChem | |
URL | https://pubchem.ncbi.nlm.nih.gov | |
Description | Data deposited in or computed by PubChem | |
InChI Key |
ATJFFYVFTNAWJD-NOHWODKXSA-N |
Source
|
Source | PubChem | |
URL | https://pubchem.ncbi.nlm.nih.gov | |
Description | Data deposited in or computed by PubChem | |
Canonical SMILES |
[Sn] |
Source
|
Source | PubChem | |
URL | https://pubchem.ncbi.nlm.nih.gov | |
Description | Data deposited in or computed by PubChem | |
Isomeric SMILES |
[112Sn] |
Source
|
Source | PubChem | |
URL | https://pubchem.ncbi.nlm.nih.gov | |
Description | Data deposited in or computed by PubChem | |
Molecular Formula |
Sn |
Source
|
Source | PubChem | |
URL | https://pubchem.ncbi.nlm.nih.gov | |
Description | Data deposited in or computed by PubChem | |
DSSTOX Substance ID |
DTXSID30893503 |
Source
|
Record name | Tin-112 | |
Source | EPA DSSTox | |
URL | https://comptox.epa.gov/dashboard/DTXSID30893503 | |
Description | DSSTox provides a high quality public chemistry resource for supporting improved predictive toxicology. | |
Molecular Weight |
111.904825 g/mol |
Source
|
Source | PubChem | |
URL | https://pubchem.ncbi.nlm.nih.gov | |
Description | Data deposited in or computed by PubChem | |
CAS No. |
15125-53-8 |
Source
|
Record name | Tin, isotope of mass 112 | |
Source | ChemIDplus | |
URL | https://pubchem.ncbi.nlm.nih.gov/substance/?source=chemidplus&sourceid=0015125538 | |
Description | ChemIDplus is a free, web search system that provides access to the structure and nomenclature authority files used for the identification of chemical substances cited in National Library of Medicine (NLM) databases, including the TOXNET system. | |
Record name | Tin-112 | |
Source | EPA DSSTox | |
URL | https://comptox.epa.gov/dashboard/DTXSID30893503 | |
Description | DSSTox provides a high quality public chemistry resource for supporting improved predictive toxicology. | |
Descargo de responsabilidad e información sobre productos de investigación in vitro
Tenga en cuenta que todos los artículos e información de productos presentados en BenchChem están destinados únicamente con fines informativos. Los productos disponibles para la compra en BenchChem están diseñados específicamente para estudios in vitro, que se realizan fuera de organismos vivos. Los estudios in vitro, derivados del término latino "in vidrio", involucran experimentos realizados en entornos de laboratorio controlados utilizando células o tejidos. Es importante tener en cuenta que estos productos no se clasifican como medicamentos y no han recibido la aprobación de la FDA para la prevención, tratamiento o cura de ninguna condición médica, dolencia o enfermedad. Debemos enfatizar que cualquier forma de introducción corporal de estos productos en humanos o animales está estrictamente prohibida por ley. Es esencial adherirse a estas pautas para garantizar el cumplimiento de los estándares legales y éticos en la investigación y experimentación.