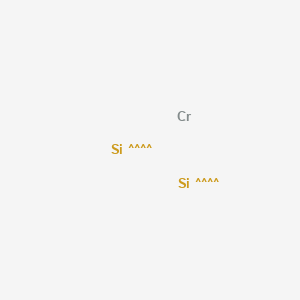
CHROMIUM SILICIDE
- Haga clic en CONSULTA RÁPIDA para recibir una cotización de nuestro equipo de expertos.
- Con productos de calidad a un precio COMPETITIVO, puede centrarse más en su investigación.
Descripción general
Descripción
CHROMIUM SILICIDE is a useful research compound. Its molecular formula is CrSi2 and its molecular weight is 108.17 g/mol. The purity is usually 95%.
BenchChem offers high-quality this compound suitable for many research applications. Different packaging options are available to accommodate customers' requirements. Please inquire for more information about this compound including the price, delivery time, and more detailed information at info@benchchem.com.
Mecanismo De Acción
Target of Action
Chromium Silicide (CrSi) primarily targets silicon (Si) atoms in the solid phase . The interaction between chromium and silicon is a key aspect of the compound’s action. Chromium in silicon has an increased reactivity , which is crucial for the formation of chromium silicides.
Mode of Action
The interaction between chromium and silicon leads to the formation of chromium silicides. This process involves the diffusion of silicon atoms in the solid phase . Chromium silicides are formed through currentless diffusion saturation, metal-thermal reduction, and electrochemical behaviors of chromium- and silicon-containing melts . The combined reduction of chromium (III) chloride and sodium fluorine-silicate by metallic sodium or magnesium makes it possible to obtain silicide powders .
Biochemical Pathways
Chromium silicides are formed through several pathways, including currentless diffusion saturation, metal-thermal reduction of the compounds of metals from groups IV–VIB and silicon with alkaline and alkaline-earth metals, and electrochemical synthesis (ES) from the melts . These processes lead to the formation of chromium silicides in the form of superfine powders and coatings .
Pharmacokinetics
The formation of chromium silicides involves the diffusion of silicon atoms in the solid phase, suggesting that the compound’s bioavailability may be influenced by this process .
Result of Action
The result of this compound’s action is the formation of chromium silicides in the form of superfine powders and coatings . These silicides are characterized by their valuable physicochemical properties and high thermal and chemical stability . They are also environmentally safe materials .
Action Environment
The action of this compound is influenced by environmental factors such as temperature and the presence of other compounds. For instance, the synthesis of chromium silicides requires high temperatures . Additionally, the presence of other compounds, such as chromium (III) chloride and sodium fluorine-silicate, influences the formation of chromium silicides .
Propiedades
Número CAS |
12018-09-6 |
---|---|
Fórmula molecular |
CrSi2 |
Peso molecular |
108.17 g/mol |
InChI |
InChI=1S/Cr.2Si |
Clave InChI |
CHXGWONBPAADHP-UHFFFAOYSA-N |
SMILES |
[Si].[Si].[Cr] |
SMILES canónico |
[Si]#[Cr]#[Si] |
Origen del producto |
United States |
Q1: How can chromium silicide be formed?
A1: this compound can be formed through various methods, including:
- Ion-beam mixing: This technique involves bombarding a chromium/silicon bilayer with argon ions, leading to the intermixing of the elements and subsequent silicide formation. []
- Thermal annealing: Heating a chromium film deposited on a silicon substrate promotes silicon diffusion into the chromium layer, resulting in silicide formation. [, , , ]
- Pack cementation: This process utilizes a powder pack containing chromium and silicon sources to deposit this compound onto a substrate at high temperatures. []
- Electrochemical synthesis: Chromium silicides can be synthesized electrochemically in molten salt systems containing chromium and silicon precursors. [, ]
- Mechanical alloying: This method uses mechanical energy to induce chemical reactions between chromium and silicon powders, ultimately forming this compound. []
Q2: Does the substrate material influence the formation of this compound?
A: Yes, the substrate material can influence the chemical composition and oxidation state of chromium within the silicide film. For instance, this compound films deposited on silicon substrates exhibit different oxide contents and chromium oxidation states compared to those deposited on glass or silicon nitride substrates. []
Q3: What happens to inert gases like krypton during this compound formation?
A: The behavior of implanted inert gases during silicide formation depends on their initial location. Krypton implanted in the chromium film remains stationary relative to the chromium during silicide growth. In contrast, krypton implanted in the silicon substrate accumulates at the interface between the silicon and the growing silicide. []
Q4: What are the common stoichiometries of this compound?
A: Several this compound phases exist, including Cr3Si, Cr5Si3, CrSi, and CrSi2. Each phase exhibits distinct structural and electronic properties, making them suitable for different applications. [, , , ]
Q5: How does the silicon content affect the properties of this compound?
A: Increasing the silicon content generally leads to changes in the material's electronic structure and bonding characteristics. For example, higher silicon content in chromium silicides correlates with: * Weaker autoionization contributions in the Cr-M2,3 VV Auger spectra []* Narrower Cr-M2,3 absorption structures that approach the edge energy []
Q6: What are the advantages of using this compound in high-temperature applications?
A: this compound exhibits high melting points and excellent oxidation resistance, making it suitable for high-temperature applications. This is particularly relevant in areas like thermoelectric energy conversion and high-temperature sensors. [, ]
Q7: How does the presence of this compound affect the performance of amorphous silicon solar cells?
A: Introducing thin layers of this compound at the interfaces between metal electrodes and doped regions in amorphous silicon solar cells can significantly enhance their performance. This improvement stems from the reduction in activation energy (~0.225 eV) observed in both n-doped and p-doped amorphous silicon films when in contact with this compound. This reduction in activation energy contributes to both built-in and open-circuit voltage enhancements. [, , ]
Q8: Can this compound enhance the stability of field emitter arrays?
A: Yes, applying a this compound coating to silicon field emitters improves their stability by reducing current fluctuations. This stabilization arises from the reduced number of chemically active sites on the silicide-protected surface, leading to a chemically stable layer with higher electrical conductivity. []
Q9: Does this compound exhibit catalytic activity?
A: While the provided research doesn't specifically focus on the catalytic properties of this compound, its presence influences chemical reactions. For instance, in the SiC-Cr-Si system, this compound formation can occur through the reduction of silica (SiO2) by silicon and silicon carbide, suggesting a potential catalytic role for this compound in this reaction. []
Q10: Have theoretical calculations been employed to study chromium silicides?
A10: Yes, first-principles calculations based on density functional theory have been used to predict various properties of chromium silicides, including:
- Chemical stability: Enthalpies and binding energies calculations provide insights into the thermodynamic stability of different this compound phases. []
- Mechanical properties: Calculations of elastic moduli, such as bulk modulus, shear modulus, and Young’s modulus, reveal the mechanical strength and stiffness of these compounds. []
- Electronic properties: Density of states calculations provide valuable information regarding the electronic band structure and bonding characteristics of chromium silicides. []
- Thermodynamic properties: The Debye temperature, related to the vibrations of atoms within the material, can be calculated using theoretical models like the Debye quasi-harmonic approximation. []
Q11: What are the environmental implications of using this compound?
A11: While the provided research doesn't directly address the environmental impact of this compound, it's crucial to consider the potential environmental implications of its production, use, and disposal. Assessing its ecotoxicological effects and developing strategies for responsible waste management and recycling are important for ensuring environmental sustainability.
Q12: Are there alternative materials to this compound for similar applications?
A: Yes, depending on the specific application, several alternative materials exist. For instance, other metal silicides, like tungsten silicide, exhibit comparable high-temperature properties and are explored for thermoelectric applications. [, ] Similarly, other materials, such as titanium nitride (TiN), are investigated as diffusion barriers in microelectronic applications. [] The choice of the most suitable material depends on factors like cost, performance requirements, and specific application needs.
Descargo de responsabilidad e información sobre productos de investigación in vitro
Tenga en cuenta que todos los artículos e información de productos presentados en BenchChem están destinados únicamente con fines informativos. Los productos disponibles para la compra en BenchChem están diseñados específicamente para estudios in vitro, que se realizan fuera de organismos vivos. Los estudios in vitro, derivados del término latino "in vidrio", involucran experimentos realizados en entornos de laboratorio controlados utilizando células o tejidos. Es importante tener en cuenta que estos productos no se clasifican como medicamentos y no han recibido la aprobación de la FDA para la prevención, tratamiento o cura de ninguna condición médica, dolencia o enfermedad. Debemos enfatizar que cualquier forma de introducción corporal de estos productos en humanos o animales está estrictamente prohibida por ley. Es esencial adherirse a estas pautas para garantizar el cumplimiento de los estándares legales y éticos en la investigación y experimentación.