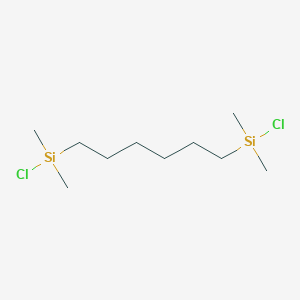
1,6-Bis(clorodimetilsilil)hexano
Descripción general
Descripción
1,6-Bis(chlorodimethylsilyl)hexane is an organosilicon compound with the molecular formula C10H24Cl2Si2. It is a bifunctional silane, meaning it contains two silicon atoms each bonded to two methyl groups and one chlorine atom. This compound is used in various chemical synthesis processes due to its reactivity and ability to form stable silicon-carbon bonds .
Aplicaciones Científicas De Investigación
1,6-Bis(chlorodimethylsilyl)hexane has several applications in scientific research and industry:
Chemistry: It is used as a precursor for the synthesis of various organosilicon compounds and as a cross-linking agent in the production of silicone polymers.
Biology: The compound is used in the modification of biomolecules to enhance their stability and reactivity.
Medicine: It is explored for use in drug delivery systems due to its ability to form stable silicon-carbon bonds.
Industry: It is used in the production of high-performance materials such as sealants, adhesives, and coatings
Mecanismo De Acción
Target of Action
1,6-Bis(chlorodimethylsilyl)hexane is an organosilicon compoundOrganosilicon compounds in general are known to interact with a variety of biological targets, depending on their specific structure and functional groups .
Mode of Action
Organosilicon compounds are known to interact with their targets through various mechanisms, including covalent bonding, hydrogen bonding, and van der waals interactions . The chlorodimethylsilyl groups in 1,6-Bis(chlorodimethylsilyl)hexane may undergo reactions with nucleophilic groups in biological targets, leading to changes in the target’s structure and function .
Biochemical Pathways
Organosilicon compounds can affect a variety of biochemical pathways, depending on their specific structure and functional groups .
Pharmacokinetics
Organosilicon compounds in general are known to have unique pharmacokinetic properties due to their silicon content . The hydrolytic sensitivity of 1,6-Bis(chlorodimethylsilyl)hexane indicates that it reacts rapidly with moisture, water, and protic solvents , which could influence its bioavailability.
Result of Action
Organosilicon compounds can have a variety of effects at the molecular and cellular level, depending on their specific structure and functional groups .
Action Environment
Environmental factors can significantly influence the action, efficacy, and stability of 1,6-Bis(chlorodimethylsilyl)hexane. For instance, the presence of moisture or water can lead to rapid hydrolysis of the compound . Additionally, the compound’s reactivity may be influenced by pH, temperature, and the presence of other chemicals in the environment .
Análisis Bioquímico
Biochemical Properties
It is known that it reacts rapidly with moisture, water, and protic solvents . This suggests that it may interact with enzymes, proteins, and other biomolecules in a biochemical context, but specific interactions have not been reported.
Cellular Effects
Given its reactivity with water and protic solvents , it may influence cell function by interacting with cellular components, but specific impacts on cell signaling pathways, gene expression, and cellular metabolism have not been documented.
Molecular Mechanism
Its potential to bind to biomolecules and influence enzyme activity or gene expression is suggested by its chemical reactivity , but specific mechanisms have not been reported.
Métodos De Preparación
Synthetic Routes and Reaction Conditions
1,6-Bis(chlorodimethylsilyl)hexane can be synthesized through the reaction of chlorodimethylsilane with 1,5-hexadiene. The reaction typically involves the following steps :
Reactants: Chlorodimethylsilane and 1,5-hexadiene.
Catalyst: A platinum-based catalyst is often used to facilitate the hydrosilylation reaction.
Reaction Conditions: The reaction is carried out under an inert atmosphere (e.g., nitrogen) to prevent oxidation. The temperature is maintained around 50-100°C.
Industrial Production Methods
In industrial settings, the production of 1,6-Bis(chlorodimethylsilyl)hexane follows similar synthetic routes but on a larger scale. The process involves continuous flow reactors to ensure efficient mixing and reaction of the reactants. The product is then purified through distillation to remove any unreacted starting materials and by-products .
Análisis De Reacciones Químicas
Types of Reactions
1,6-Bis(chlorodimethylsilyl)hexane undergoes several types of chemical reactions, including:
Substitution Reactions: The chlorine atoms can be replaced by other nucleophiles such as alkoxides, amines, or thiols.
Hydrolysis: The compound reacts rapidly with water to form silanols and hydrochloric acid.
Polymerization: It can be used as a cross-linking agent in the formation of silicone polymers.
Common Reagents and Conditions
Substitution Reactions: Common reagents include sodium alkoxides, primary or secondary amines, and thiols. The reactions are typically carried out in anhydrous solvents such as tetrahydrofuran (THF) or toluene.
Hydrolysis: This reaction occurs readily in the presence of moisture or water.
Polymerization: Catalysts such as tin or platinum compounds are used to initiate the polymerization process.
Major Products Formed
Substitution Reactions: The major products are the corresponding substituted silanes.
Hydrolysis: The primary products are silanols and hydrochloric acid.
Polymerization: The major products are silicone polymers with varying degrees of cross-linking.
Comparación Con Compuestos Similares
Similar Compounds
1,6-Bis(trimethylsilyl)hexane: Similar structure but with trimethylsilyl groups instead of chlorodimethylsilyl groups.
1,6-Bis(dimethylsilyl)hexane: Lacks the chlorine atoms, making it less reactive in substitution reactions.
1,6-Bis(methyldichlorosilyl)hexane: Contains two chlorine atoms on each silicon, making it more reactive than 1,6-Bis(chlorodimethylsilyl)hexane.
Uniqueness
1,6-Bis(chlorodimethylsilyl)hexane is unique due to its bifunctional nature, allowing it to participate in a wide range of chemical reactions. Its reactivity, particularly in substitution reactions, makes it a valuable intermediate in the synthesis of various organosilicon compounds .
Actividad Biológica
1,6-Bis(chlorodimethylsilyl)hexane (CAS Number: 14799-66-7) is an organosilicon compound characterized by its bifunctional silane structure, comprising two silicon atoms bonded to methyl groups and chlorine atoms. This compound has garnered interest in various fields, particularly in chemistry, biology, and material science due to its potential biological activities and utility in synthesizing silicone-based materials.
1,6-Bis(chlorodimethylsilyl)hexane has the molecular formula C10H24Cl2Si2. Its reactivity is influenced by the presence of chlorine atoms, which can participate in substitution reactions with nucleophiles. The compound is known to hydrolyze readily in the presence of moisture, producing silanols and hydrochloric acid. This property is critical for its applications in modifying biomolecules and creating stable silicon-carbon bonds.
The biological activity of organosilicon compounds like 1,6-bis(chlorodimethylsilyl)hexane is often attributed to their ability to interact with biological targets through several mechanisms:
- Covalent Bonding: The chlorine atoms can form covalent bonds with nucleophilic sites on biomolecules.
- Hydrogen Bonding: The presence of silanol groups can facilitate hydrogen bonding with proteins and nucleic acids.
- Van der Waals Interactions: These interactions contribute to the stabilization of molecular complexes.
Cytotoxicity and Anticancer Activity
Preliminary investigations into the cytotoxic effects of organosilicon compounds have shown promise in anticancer applications. For instance, compounds that can modify cellular pathways or inhibit enzyme activity have been explored for their potential to induce apoptosis in cancer cells. The reactivity of 1,6-bis(chlorodimethylsilyl)hexane may allow it to interact with key cellular targets involved in cancer progression.
Biochemical Pathways
The interaction of 1,6-bis(chlorodimethylsilyl)hexane with biological systems can influence various biochemical pathways:
- Enzyme Modulation: By binding to active sites on enzymes, it may alter their activity.
- Gene Expression: Its potential to affect gene expression through interactions with DNA or RNA could have implications for gene therapy applications.
Pharmacokinetics
The pharmacokinetic profile of organosilicon compounds is unique due to their silicon content. Factors such as absorption, distribution, metabolism, and excretion (ADME) can vary significantly compared to traditional organic compounds. The rapid hydrolysis of 1,6-bis(chlorodimethylsilyl)hexane suggests that its bioavailability might be influenced by environmental conditions within biological systems.
Case Studies
While direct studies on 1,6-bis(chlorodimethylsilyl)hexane are sparse, related research provides insight into its potential applications:
- Synthesis of Silica Gels: In studies involving polyhalosilanes as cross-linking agents for silica gels, the incorporation of similar organosilicon compounds has demonstrated improved mechanical properties and stability under physiological conditions.
- Drug Delivery Systems: Research has explored the use of silanes in drug delivery systems due to their ability to form stable complexes with therapeutic agents.
Propiedades
IUPAC Name |
chloro-[6-[chloro(dimethyl)silyl]hexyl]-dimethylsilane | |
---|---|---|
Source | PubChem | |
URL | https://pubchem.ncbi.nlm.nih.gov | |
Description | Data deposited in or computed by PubChem | |
InChI |
InChI=1S/C10H24Cl2Si2/c1-13(2,11)9-7-5-6-8-10-14(3,4)12/h5-10H2,1-4H3 | |
Source | PubChem | |
URL | https://pubchem.ncbi.nlm.nih.gov | |
Description | Data deposited in or computed by PubChem | |
InChI Key |
SEEQROYMPAMQOF-UHFFFAOYSA-N | |
Source | PubChem | |
URL | https://pubchem.ncbi.nlm.nih.gov | |
Description | Data deposited in or computed by PubChem | |
Canonical SMILES |
C[Si](C)(CCCCCC[Si](C)(C)Cl)Cl | |
Source | PubChem | |
URL | https://pubchem.ncbi.nlm.nih.gov | |
Description | Data deposited in or computed by PubChem | |
Molecular Formula |
C10H24Cl2Si2 | |
Source | PubChem | |
URL | https://pubchem.ncbi.nlm.nih.gov | |
Description | Data deposited in or computed by PubChem | |
DSSTOX Substance ID |
DTXSID50373493 | |
Record name | 1,6-BIS(CHLORODIMETHYLSILYL)HEXANE | |
Source | EPA DSSTox | |
URL | https://comptox.epa.gov/dashboard/DTXSID50373493 | |
Description | DSSTox provides a high quality public chemistry resource for supporting improved predictive toxicology. | |
Molecular Weight |
271.37 g/mol | |
Source | PubChem | |
URL | https://pubchem.ncbi.nlm.nih.gov | |
Description | Data deposited in or computed by PubChem | |
CAS No. |
14799-66-7 | |
Record name | 1,6-BIS(CHLORODIMETHYLSILYL)HEXANE | |
Source | EPA DSSTox | |
URL | https://comptox.epa.gov/dashboard/DTXSID50373493 | |
Description | DSSTox provides a high quality public chemistry resource for supporting improved predictive toxicology. | |
Retrosynthesis Analysis
AI-Powered Synthesis Planning: Our tool employs the Template_relevance Pistachio, Template_relevance Bkms_metabolic, Template_relevance Pistachio_ringbreaker, Template_relevance Reaxys, Template_relevance Reaxys_biocatalysis model, leveraging a vast database of chemical reactions to predict feasible synthetic routes.
One-Step Synthesis Focus: Specifically designed for one-step synthesis, it provides concise and direct routes for your target compounds, streamlining the synthesis process.
Accurate Predictions: Utilizing the extensive PISTACHIO, BKMS_METABOLIC, PISTACHIO_RINGBREAKER, REAXYS, REAXYS_BIOCATALYSIS database, our tool offers high-accuracy predictions, reflecting the latest in chemical research and data.
Strategy Settings
Precursor scoring | Relevance Heuristic |
---|---|
Min. plausibility | 0.01 |
Model | Template_relevance |
Template Set | Pistachio/Bkms_metabolic/Pistachio_ringbreaker/Reaxys/Reaxys_biocatalysis |
Top-N result to add to graph | 6 |
Feasible Synthetic Routes
Descargo de responsabilidad e información sobre productos de investigación in vitro
Tenga en cuenta que todos los artículos e información de productos presentados en BenchChem están destinados únicamente con fines informativos. Los productos disponibles para la compra en BenchChem están diseñados específicamente para estudios in vitro, que se realizan fuera de organismos vivos. Los estudios in vitro, derivados del término latino "in vidrio", involucran experimentos realizados en entornos de laboratorio controlados utilizando células o tejidos. Es importante tener en cuenta que estos productos no se clasifican como medicamentos y no han recibido la aprobación de la FDA para la prevención, tratamiento o cura de ninguna condición médica, dolencia o enfermedad. Debemos enfatizar que cualquier forma de introducción corporal de estos productos en humanos o animales está estrictamente prohibida por ley. Es esencial adherirse a estas pautas para garantizar el cumplimiento de los estándares legales y éticos en la investigación y experimentación.