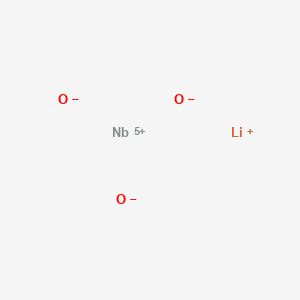
Lithium niobium trioxide
Descripción general
Descripción
Lithium niobium trioxide (LiNbO₃), with the CAS number 12031-63-9, is an inorganic compound composed of lithium, niobium, and oxygen . It is a solid at room temperature, with a melting point of 1275°C, and is primarily used in laboratory settings and chemical manufacturing . LiNbO₃ is notable for its applications in optoelectronics, piezoelectric devices, and lithium-ion battery research due to its ferroelectric and nonlinear optical properties . However, it poses specific hazards, including acute oral toxicity (H302), skin irritation (H315), and serious eye irritation (H319) .
Métodos De Preparación
Synthetic Routes and Reaction Conditions: Lithium niobium trioxide can be synthesized through several methods. One common method involves the reaction of lithium carbonate with niobium pentoxide in a platinum crucible. The mixture is heated to a high temperature, typically around 1240°C, to form this compound crystals . Another method involves the thermal decomposition of an organic niobium salt and lithium nitrate in a buffer solution of oxalic acid and ammonium oxalate. The resulting precursor is then heat-treated to yield this compound .
Industrial Production Methods: In industrial settings, this compound is often produced using the Czochralski process. This method involves melting the raw materials in a crucible and then slowly pulling a seed crystal from the melt to form a large single crystal. The crystal is then sliced into wafers for various applications .
Análisis De Reacciones Químicas
Types of Reactions: Lithium niobium trioxide undergoes several types of chemical reactions, including oxidation, reduction, and substitution reactions.
Common Reagents and Conditions:
Oxidation: this compound can be oxidized using strong oxidizing agents such as hydrogen peroxide or potassium permanganate.
Reduction: Reduction reactions can be carried out using reducing agents like lithium hydride or sodium borohydride.
Major Products Formed: The major products formed from these reactions depend on the specific reagents and conditions used. For example, oxidation may yield higher oxidation state niobium compounds, while reduction may produce lower oxidation state niobium compounds .
Aplicaciones Científicas De Investigación
Energy Storage Applications
Lithium-ion Batteries (LIBs)
Lithium niobium trioxide is increasingly utilized in lithium-ion batteries as a cathode material due to its favorable electrochemical properties. It serves as both a dopant and a coating material, enhancing the performance of lithium transition metal oxides (LTMOs).
- Doping Effects : Niobium doping improves the cycling stability and rate capability of cathodes. For instance, studies indicate that Nb-doped exhibits reduced voltage decay and enhanced stability at high rates .
- Coating Benefits : Coatings of on cathodes have shown to decrease first-cycle loss and improve lithium diffusion kinetics, which is critical for high-performance batteries .
Table 1: Performance Metrics of Nb-Doped Cathodes
Cathode Material | Nb Doping Level | Cycle Stability | Rate Capability |
---|---|---|---|
2% | High | Excellent | |
5% | Moderate | Good | |
3% | High | Very Good |
Electronics and Photonics
Nonlinear Optical Devices
The unique optical properties of make it suitable for applications in nonlinear optics, such as frequency doubling and optical waveguides. Its ability to generate second harmonic generation (SHG) has been exploited in various laser technologies.
- Case Study : A notable application involves the use of in optical modulators for telecommunications, where it aids in signal processing through electro-optic effects .
Materials Science
Tunable Materials
This compound is explored as a tunable material in various scientific fields due to its ferroelectric properties. This characteristic allows it to be used in capacitors and sensors.
- Research Findings : Studies have demonstrated that the ferroelectric properties of can be modified through doping, leading to enhanced performance in piezoelectric devices .
Neuromorphic Computing
Recent advancements have highlighted the potential of in neuromorphic computing applications. Its ability to mimic synaptic functions makes it a candidate for developing brain-inspired computing systems.
Mecanismo De Acción
The mechanism of action of lithium niobium trioxide is primarily based on its ferroelectric and piezoelectric properties. These properties arise from the alignment of electric dipoles within the crystal structure. When an electric field is applied, the dipoles align, resulting in a change in the material’s polarization. This change can be harnessed for various applications, such as signal modulation in optical devices and sensing in piezoelectric sensors .
Comparación Con Compuestos Similares
Lithium niobium trioxide belongs to a broader class of lithium transition metal oxides and niobium-based compounds. Below is a detailed comparison with structurally or functionally analogous materials:
Table 1: Comparative Analysis of LiNbO₃ and Similar Compounds
Key Comparisons
Structural and Thermal Stability :
LiNbO₃ and LiTaO₃ share a perovskite-like structure, but LiTaO₃ exhibits higher thermal stability (melting point ~1650°C vs. 1275°C for LiNbO₃) . This makes LiTaO₃ preferable in high-temperature applications like surface acoustic wave devices.- Electrochemical Applications: While LiNbO₃ is explored for solid-state electrolytes, LiCoO₂ dominates as a commercial cathode material in lithium-ion batteries due to higher energy density. However, LiCoO₂ carries carcinogenic risks (H350), unlike LiNbO₃ .
Hazard Profiles :
LiNbO₃’s primary hazards (oral toxicity, skin/eye irritation) differ from Nb₂O₅, which lacks acute toxicity classifications in the provided sources . Lithium oxide (Li₂O), by contrast, poses severe corrosion risks (H314) due to its reactivity with water .Niobium-Based Compounds : Nb₂O₅ is widely used in catalysis and sensors but lacks the ferroelectric properties of LiNbO₃. Its lower hazard profile makes it safer for industrial use .
Q & A
Basic Research Questions
Q. What are the standard synthesis methods for LiNbO₃ single crystals, and how does non-stoichiometry affect crystal growth?
LiNbO₃ is typically synthesized via the Czochralski method for single crystals or solid-state reactions for polycrystalline forms. Congruent LiNbO₃ (non-stoichiometric) exhibits excess niobium occupying lithium sites, compensated by lithium vacancies (4 vacancies per Nb antisite defect) . Methodological steps :
- Control atmosphere (oxygen-rich) to minimize defects.
- Optimize temperature gradients during crystal pulling to reduce stress.
- Post-growth annealing to homogenize vacancy distribution.
Stoichiometric LiNbO₃ requires vapor transport equilibration (VTE) to adjust Li/Nb ratios .
Q. What safety protocols are critical for handling LiNbO₃ in laboratory settings?
LiNbO₃ requires precautions due to its acute toxicity (H302) and respiratory irritation (H335) :
- Use gloves (nitrile) and safety goggles to avoid skin/eye contact.
- Employ local exhaust ventilation to minimize dust inhalation.
- Store in dry, airtight containers at room temperature to prevent hydrolysis.
- Characterize purity via XRD/EDS before use to avoid experimental artifacts .
Q. Which characterization techniques are essential for verifying LiNbO₃ stoichiometry and defect structures?
- XRD : Confirms crystal phase and lattice parameters (e.g., shifts in peaks indicate Li/Nb ratio changes) .
- Raman Spectroscopy : Identifies defect-related phonon modes (e.g., Nb antisite defects at ~630 cm⁻¹) .
- Positron Annihilation Spectroscopy : Quantifies lithium vacancy concentration .
- Electron Paramagnetic Resonance (EPR) : Detects paramagnetic dopants (e.g., V²⁺, Mo³⁺) and their lattice sites .
Advanced Research Questions
Q. How do variations in lithium diffusion coefficients (D) and activation energy (E) impact ion transport mechanisms?
Reported D ranges from 1.5×10⁻⁹ to 5.7×10³ cm²/s, with E spanning 0.36–3.24 eV due to non-stoichiometry and measurement techniques . Methodological resolution :
- Use impedance spectroscopy for bulk conductivity vs. tracer diffusion (e.g., Li⁶ isotope) for direct diffusion measurement.
- Apply Arrhenius analysis to decouple vacancy-mediated vs. interstitial diffusion pathways.
- Compare congruent vs. stoichiometric crystals to isolate defect effects .
Q. What computational strategies model dopant incorporation in LiNbO₃, and how do they align with experiments?
Density Functional Theory (DFT) simulates dopant energetics:
- V²⁺ favors Li sites with vacancy compensation, while V³⁺/Mo³⁺ self-compensate via dual-site occupation .
Validation steps : - Cross-check simulated defect formation energies with EPR (site occupancy) and XPS (oxidation states).
- Correlate calculated bandgap shifts with UV-Vis absorption spectra .
Q. How can thermal stability of LiNbO₃ be optimized for ultra-high temperature applications (e.g., heat pipes)?
Design considerations from Nb-1%Zr alloy heat pipes (~1700 K operation) :
- Wick structure : 7-layer 400-mesh annulus to reduce thermal resistance.
- Vapor chamber : Diameter optimized for Mach number <0.2 to prevent choking.
- Validation : Compare FLUENT simulations (vapor pressure, temp gradients) with experimental steady-state tests .
Q. How do charge compensation mechanisms (vacancies vs. electronic) influence LiNbO₃'s optoelectronic properties?
- Lithium vacancy compensation (4 Vₗᵢ per Nb antisite) increases ionic conductivity but introduces optical absorption centers .
- Electronic compensation (e.g., polaronic hopping) enhances photoconductivity but reduces piezoelectric response.
Experimental differentiation : - Measure AC impedance (ionic vs. electronic contributions).
- Use UV-Vis-NIR spectroscopy to track defect-related absorption bands .
Propiedades
IUPAC Name |
lithium;niobium(5+);oxygen(2-) | |
---|---|---|
Source | PubChem | |
URL | https://pubchem.ncbi.nlm.nih.gov | |
Description | Data deposited in or computed by PubChem | |
InChI |
InChI=1S/Li.Nb.3O/q+1;+5;3*-2 | |
Source | PubChem | |
URL | https://pubchem.ncbi.nlm.nih.gov | |
Description | Data deposited in or computed by PubChem | |
InChI Key |
PSVBHJWAIYBPRO-UHFFFAOYSA-N | |
Source | PubChem | |
URL | https://pubchem.ncbi.nlm.nih.gov | |
Description | Data deposited in or computed by PubChem | |
Canonical SMILES |
[Li+].[O-2].[O-2].[O-2].[Nb+5] | |
Source | PubChem | |
URL | https://pubchem.ncbi.nlm.nih.gov | |
Description | Data deposited in or computed by PubChem | |
Molecular Formula |
LiNbO3 | |
Source | PubChem | |
URL | https://pubchem.ncbi.nlm.nih.gov | |
Description | Data deposited in or computed by PubChem | |
Related CAS |
12034-89-8 (strontium(2:1) salt), 12059-51-7 (rubidium salt) | |
Record name | Lithium niobate | |
Source | ChemIDplus | |
URL | https://pubchem.ncbi.nlm.nih.gov/substance/?source=chemidplus&sourceid=0012031639 | |
Description | ChemIDplus is a free, web search system that provides access to the structure and nomenclature authority files used for the identification of chemical substances cited in National Library of Medicine (NLM) databases, including the TOXNET system. | |
Molecular Weight |
147.9 g/mol | |
Source | PubChem | |
URL | https://pubchem.ncbi.nlm.nih.gov | |
Description | Data deposited in or computed by PubChem | |
CAS No. |
12031-63-9 | |
Record name | Lithium niobate | |
Source | ChemIDplus | |
URL | https://pubchem.ncbi.nlm.nih.gov/substance/?source=chemidplus&sourceid=0012031639 | |
Description | ChemIDplus is a free, web search system that provides access to the structure and nomenclature authority files used for the identification of chemical substances cited in National Library of Medicine (NLM) databases, including the TOXNET system. | |
Descargo de responsabilidad e información sobre productos de investigación in vitro
Tenga en cuenta que todos los artículos e información de productos presentados en BenchChem están destinados únicamente con fines informativos. Los productos disponibles para la compra en BenchChem están diseñados específicamente para estudios in vitro, que se realizan fuera de organismos vivos. Los estudios in vitro, derivados del término latino "in vidrio", involucran experimentos realizados en entornos de laboratorio controlados utilizando células o tejidos. Es importante tener en cuenta que estos productos no se clasifican como medicamentos y no han recibido la aprobación de la FDA para la prevención, tratamiento o cura de ninguna condición médica, dolencia o enfermedad. Debemos enfatizar que cualquier forma de introducción corporal de estos productos en humanos o animales está estrictamente prohibida por ley. Es esencial adherirse a estas pautas para garantizar el cumplimiento de los estándares legales y éticos en la investigación y experimentación.