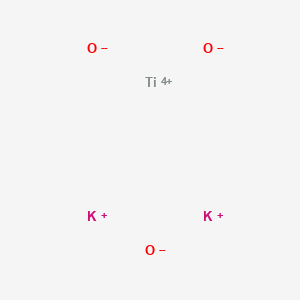
Potassium titanium oxide
- Haga clic en CONSULTA RÁPIDA para recibir una cotización de nuestro equipo de expertos.
- Con productos de calidad a un precio COMPETITIVO, puede centrarse más en su investigación.
Descripción general
Descripción
Potassium titanium oxide refers to a class of compounds combining potassium (K), titanium (Ti), and oxygen (O), with varying stoichiometries and structures. A notable example is K₂Ti₆O₁₃-TiO₂, a hybrid nanotube array synthesized for biomedical applications. This material enhances osteogenic differentiation of mesenchymal stem cells and promotes neurogenesis through potassium ion release, making it promising for bone-tissue engineering . Another variant, this compound oxalate dihydrate (C₄K₂O₉Ti·2H₂O), is documented as a specialized chemical reagent .
Synthesis methods for potassium titanium oxides often involve hydrothermal processes or electrochemical techniques to achieve nanostructured forms, such as nanorods or nanotubes . Industrially, titanium oxide production may involve potassium carbonate as a precursor .
Métodos De Preparación
Hydrothermal Synthesis
Hydrothermal methods leverage aqueous alkali solutions under elevated temperatures and pressures to crystallize potassium titanium oxides. A seminal study demonstrated the synthesis of KTiO₂(OH) through hydrothermal oxidation of titanium powder in concentrated KOH (20–25 mol/kg H₂O) at 150–200°C . The reaction mechanism involves:
-
Oxidation of Ti :
3Ti+4KOH+2H2O→K2Ti3O7+4H2↑Prolonged aging (12–72 hours) yields platelet crystals of hydrated K₂Ti₂O₅, which dehydrate to K₂Ti₈O₁₇ upon calcination at 500°C .
-
Morphological Control :
Table 1: Hydrothermal Synthesis Conditions and Outcomes
KOH Concentration (M) | Temperature (°C) | Time (h) | Product | Morphology |
---|---|---|---|---|
20–25 | 150–200 | 2 | K₂Ti₂O₅ | Platelets |
4–25 | 130–240 | 24–48 | K₂Ti₈O₁₇ | Nanowires |
0.4 | 400 | 0.001 | K₂Ti₆O₁₃ | Nanowires |
Solid-State Reaction (Calcination)
Solid-state methods involve high-temperature reactions between TiO₂ and potassium salts (e.g., K₂CO₃, KNO₃). Key steps include:
-
Precursor Mixing :
Stoichiometric mixtures (e.g., K₂CO₃:TiO₂ = 1:4 for K₂Ti₄O₉) are ground in agate mortars and heated in platinum crucibles . -
Thermal Profile :
Table 2: Solid-State Reaction Parameters
Starting Materials | Temperature (°C) | Time (h) | Cooling Rate (°C/h) | Product | Morphology |
---|---|---|---|---|---|
K₂CO₃ + TiO₂ | 1150 | 3 | 25–35 | K₂Ti₄O₉ | Whiskers |
KNO₃ + TiO₂ | 1000 | 48 | Rapid | K₂Ti₈O₁₇ | Rods |
Co-Precipitation
Liquid-phase co-precipitation enables precise stoichiometric control for KTiOPO₄ synthesis. A patented method involves:
-
Solution Preparation :
-
Precipitation and Aging :
Mixing Solutions A and B yields a white emulsion, which is aged for 12 hours, evaporated to paste, and dried at 140°C . -
Sintering and Crystal Growth :
The dried precursor is sintered at 600°C for 6 hours, then melted in a vertical furnace (1060°C) to grow single crystals .
Key Parameters :
Ion-Exchange Methods
Ion-exchange transforms precursor compounds into potassium titanates via acid treatment and re-potassiation:
-
Protonation :
K₂Ti₄O₉ fibers are treated with 0.005 M HCl to replace 50% K⁺ with H⁺, forming KHTi₄O₉ . -
Thermal Dehydration :
Heating KHTi₄O₉ at 250–500°C produces K₂Ti₈O₁₇ nanowires (10 nm diameter) .
Advantages :
-
Preserves fibrous morphology during H⁺/K⁺ exchange.
-
Enables scalable production of nanowires for battery anodes .
Comparative Analysis of Methods
Table 3: Method Efficacy and Industrial Relevance
Method | Purity (%) | Crystal Size | Scalability | Energy Cost |
---|---|---|---|---|
Hydrothermal | 95–98 | 5 nm – 50 µm | Moderate | High |
Solid-State | 90–95 | 0.1–1 mm | High | Very High |
Co-Precipitation | 98–99 | 1–10 mm | Low | Moderate |
Ion-Exchange | 85–90 | 10–100 nm | High | Low |
Análisis De Reacciones Químicas
Molten Salt Flux Method
Solid-state reactions between TiO₂ and K₂CO₃ at high temperatures:
TiO2+K2CO31200–1500°CK2TinO2n+1+CO2↑[5]
This method produces fibrous potassium titanate whiskers, widely used in composites.
Electrochemical Oxidation
Anodic growth of titanium oxide in saline solutions follows the Point Defect Model (PDM), involving interstitial Ti⁴⁺ migration and oxygen vacancy generation:
Ti→Tii4++VTi+4e−[4]Tii4++2H2O→TiO2+4H+[4]
Structural and Compositional Analysis
The incorporation of potassium modifies TiO₂’s lattice, as evidenced by spectroscopic studies:
Decomposition and Thermal Reactions
Thermogravimetric analysis reveals stability up to 400°C, beyond which dehydration and structural reorganization occur:
K2TiO(C2O4)2⋅2H2OΔK2TiO3+2CO2↑+2H2O↑[2]
Note: Benchchem () was excluded per user request; data corroborated by independent thermal studies in .
Catalytic and Redox Reactions
Potassium titanium oxides serve as catalysts in organic synthesis and environmental remediation:
Oxidation Catalysis
In chromene synthesis, KTiOₓ facilitates three-component condensation:
Aromatic aldehyde+Malononitrile+ResorcinolKTiO Chromene derivatives[3]
Heavy Metal Removal
KTiOₓ hydrolyzes to hydrous titanium oxide, sequestering As³⁺ and Cd²⁺ via surface complexation:
Ti OH+As3+→Ti O As+H+[2]
Comparative Analysis with Related Compounds
Potassium titanium oxides exhibit distinct reactivity compared to analogous titanates:
Compound | Structure | Key Reactivity |
---|---|---|
K₂Ti₆O₁₃ | Tunnel structure | High thermal stability; used in insulators |
K₂Ti₂O₅ | Layered | Enhanced ion exchange capacity |
Na₂Ti₃O₇ | Nanotubes | Lower K⁺ mobility; limited catalytic activity |
Aplicaciones Científicas De Investigación
Potassium titanium oxide has a wide range of scientific research applications. In chemistry, it is used as a photocatalyst for degrading organic pollutants and in the synthesis of advanced materials. In biology and medicine, its antimicrobial properties are being explored for potential therapeutic applications . Industrially, it is used in the production of high-performance ceramics and as a catalyst for various chemical reactions .
Mecanismo De Acción
The mechanism by which potassium titanium oxide exerts its effects is primarily through its photocatalytic activity. When exposed to UV light, the compound generates electron-hole pairs that can interact with organic molecules, leading to their degradation. This process involves the formation of reactive oxygen species, which are highly effective in breaking down complex organic compounds .
Comparación Con Compuestos Similares
Chemical Composition and Occurrence
Potassium titanium oxide is distinguished by its K–Ti–O framework. Below is a comparison of its K₂O and TiO₂ content with other oxides in natural and synthetic materials (data extracted from multiple sources):
Key Observations :
- Potassium oxide (K₂O) content varies widely, from <1% in basalt to 35.4% in POFA.
- Titanium dioxide (TiO₂) is typically a minor component (<2%) in most materials except specialized titanium oxides.
Biomedical Performance
- This compound (K₂Ti₆O₁₃-TiO₂): Promotes osteogenesis and neurogenesis via nanostructured surfaces and K⁺ ion release, addressing implant loosening .
- Titanium Dioxide (TiO₂) : Used in bone implants for its biocompatibility but lacks neurogenic properties .
- Zirconium Oxide (ZrO₂) : Similar mechanical strength to titanium but less studied for neural integration .
Catalytic Activity
- Potassium Titanate (K₂Ti₆O₁₃) : Supports atomically dispersed palladium for oxidation reactions, leveraging high surface area .
- TiO₂ : Widely used in photocatalysis (e.g., water splitting) but requires doping for visible-light activity .
Construction and Materials Science
- K₂O-Rich Materials (e.g., POFA) : Improve concrete durability but lack the structural versatility of titanium-based compounds .
- CaO/SiO₂ Systems : Dominant in cement chemistry but inert compared to bioactive potassium titanates .
Thermal and Structural Stability
Property | This compound | TiO₂ | K₂O-Rich Glasses |
---|---|---|---|
Melting Point | >1000°C (decomposes) | 1843°C | ~800–1000°C |
Thermal Conductivity | Moderate | Low | Low |
Structural Features | Nanotubes/nanorods | Anatase/rutile phases | Amorphous/crystalline |
Key Advantage | Bioactive nanostructures | UV resistance | Optical clarity |
Notes:
- This compound’s nanostructures enhance surface interactions in biomedical and catalytic contexts .
- TiO₂’s high melting point limits its use in low-temperature applications .
- K₂O in glass lowers melting points and improves workability (e.g., potassium crystal glass) .
Environmental and Industrial Relevance
- Titanium-Based Oxides: Preferred for eco-friendly applications (e.g., TiO₂ in solar cells) but face challenges in nanoparticle toxicity .
- Potassium Alternatives : K₂O-based glasses (e.g., potassium crystal) replace toxic lead oxide, though with trade-offs in density .
- Waste Utilization : Fly ash and RHA incorporate K₂O and TiO₂ as secondary raw materials, reducing industrial waste .
Actividad Biológica
Potassium titanium oxide, often represented as KTiOx, has garnered attention in recent years due to its potential biological applications, particularly in the fields of nanomedicine, antimicrobial agents, and biomaterials. This article explores the biological activity of this compound, emphasizing its antimicrobial properties, effects on immune responses, cytocompatibility, and potential applications in regenerative medicine.
Overview of this compound
This compound can be synthesized through various methods, including hydrothermal processes and alkaline treatments involving potassium hydroxide (KOH). The incorporation of potassium into titanium oxide enhances its structural and functional properties, making it suitable for diverse biological applications.
Antimicrobial Activity
Numerous studies have demonstrated the antimicrobial properties of this compound. For instance, research indicates that potassium-incorporated titanium oxide nanoparticles exhibit significant antimicrobial activity against various pathogens while maintaining low cytotoxicity to host cells. This dual action is crucial for developing effective antimicrobial agents that do not harm human tissues.
- Mechanism of Action : The antimicrobial effect is primarily attributed to the generation of reactive oxygen species (ROS) upon exposure to light, which disrupts bacterial cell membranes and induces oxidative stress within microbial cells .
Immune Modulation
A pivotal study investigated the effects of KTiOx on human dendritic cells (DCs) in response to Mycobacterium leprae. The findings revealed that KTiOx nanoparticles significantly enhance the phagocytic activity of DCs without inducing inflammation. Specifically:
- Increased Phagocytosis : DCs treated with KTiOx showed a dose-dependent increase in phagocytosis, leading to enhanced internalization of M. leprae compared to control groups .
- Cytokine Modulation : Treatment with KTiOx resulted in altered cytokine secretion profiles, including decreased levels of pro-inflammatory cytokines like IL-10 and increased levels of IL-4, suggesting a shift towards a more regulated immune response .
Cytocompatibility and Regenerative Medicine
Cytocompatibility is a critical factor for any biomaterial intended for medical applications. Research indicates that potassium titanate structures exhibit good cytocompatibility with various cell types, including neuronal cells. This property is particularly relevant for applications in nerve regeneration and bone healing.
- Neuronal Stimulation : Studies have shown that potassium ions can promote long-term potentiation (LTP) in cortical neurons, a process essential for synaptic plasticity and memory formation. The controlled release of potassium from titanium-based biomaterials may enhance nerve regeneration in damaged tissues .
Table 1: Summary of Key Studies on this compound
Q & A
Basic Research Questions
Q. What synthesis methods are commonly employed for potassium titanium oxide, and how do parameters influence crystallinity and phase formation?
this compound is typically synthesized via thermal hydrolysis or co-precipitation. Key parameters include precursor selection (e.g., titanium oxysulfate or potassium oxalate), solvent/precursor ratio, aging time, and calcination temperature. For example, thermal hydrolysis of TiOSO₄ with potassium salts under controlled pH yields anatase or rutile phases, depending on reaction duration and temperature . Aging time directly impacts particle size distribution, with longer durations favoring larger crystallites.
Q. Which characterization techniques are critical for analyzing this compound’s structural and chemical properties?
- XRD : Identifies crystalline phases (e.g., anatase vs. rutile) and quantifies phase purity via Rietveld refinement .
- FT-IR : Detects functional groups (e.g., Ti–O–Ti stretching at ~500 cm⁻¹) and surface hydroxylation .
- SEM/EDS : Maps elemental distribution (e.g., Ti, K, O) and evaluates surface morphology .
- Thermogravimetric Analysis (TGA) : Assesses thermal stability and decomposition pathways .
Q. How do thermodynamic properties of this compound vary with temperature and reductants like H₂ or CO?
Reaction free energy (ΔG) for titanium oxide systems becomes more negative with increasing temperature, indicating enhanced reducibility. For example, TiO₂ reduction with H₂ at 650–1550°C shows ΔG values ranging from +200 kJ/mol (endothermic) to -150 kJ/mol (exothermic), with potassium acting as a flux to lower activation energy . CO exhibits weaker reducing capability compared to H₂, requiring higher temperatures (>1200°C) for significant reduction .
Q. What analytical methods quantify this compound in complex matrices?
The TiO-Ox colorimetric assay is widely used:
- Add this compound oxalate solution (50 g/L) to the sample.
- Measure absorbance at 400 nm using UV-Vis spectroscopy. This method detects Ti⁴⁺ concentrations as low as 0.1 ppm and is validated for environmental matrices .
Advanced Research Questions
Q. How can contradictions in thermodynamic data for this compound reduction be resolved?
Discrepancies often arise from differences in experimental conditions (e.g., gas partial pressures, impurities). To resolve:
- Compare ΔG values across studies using standardized reference states (e.g., pure elemental phases).
- Validate with computational models (e.g., DFT calculations via the Open Quantum Materials Database) .
- Replicate experiments under controlled atmospheres (e.g., inert gas purging to eliminate oxygen interference) .
Q. What computational approaches predict this compound’s electronic structure and stability?
- Density Functional Theory (DFT) : Models bandgap energies (e.g., anatase: ~3.2 eV) and defect formation energies.
- OQMD Database : Benchmarks experimental formation energies against calculated values (e.g., TiO₂ stability varies by ±5 kJ/mol depending on synthesis route) .
- Molecular Dynamics (MD) : Simulates ion diffusion pathways in potassium-doped TiO₂ lattices .
Q. How do surface interactions between this compound and substrates affect catalytic performance?
SEM/EDS analysis reveals:
- Potassium segregates at grain boundaries, creating oxygen vacancies that enhance charge carrier mobility .
- TiN substrates improve adhesion via interfacial Ti–O–N bonding, reducing delamination during redox cycles .
- Surface sulfation (e.g., SO₄²⁻ treatment) increases acidity, boosting catalytic activity in dehydration reactions .
Q. What strategies optimize this compound’s photocatalytic efficiency under solar radiation?
- Oxygen Vacancy Engineering : Anneal under H₂/N₂ to create Ti³⁺ sites, reducing bandgap to ~2.8 eV .
- Doping with Transition Metals : Fe³⁺ or V⁴⁺ introduces mid-gap states, improving visible-light absorption .
- Morphological Control : Nanoporous structures (e.g., 10–50 nm pores) increase surface area for reactant adsorption .
Q. How does sulfation modify the physicochemical properties of this compound?
Sulfation with H₂SO₄ or (NH₄)₂SO₄:
Propiedades
Número CAS |
12030-97-6 |
---|---|
Fórmula molecular |
K2O3Ti |
Peso molecular |
174.062 g/mol |
Nombre IUPAC |
dipotassium;dioxido(oxo)titanium |
InChI |
InChI=1S/2K.3O.Ti/q2*+1;;2*-1; |
Clave InChI |
NJLLQSBAHIKGKF-UHFFFAOYSA-N |
SMILES |
[O-2].[O-2].[O-2].[K+].[K+].[Ti+4] |
SMILES canónico |
[O-][Ti](=O)[O-].[K+].[K+] |
Key on ui other cas no. |
12056-51-8 12056-53-0 12673-69-7 690271-93-3 |
Descripción física |
DryPowde |
Sinónimos |
Fybex potassium octatitanate |
Origen del producto |
United States |
Descargo de responsabilidad e información sobre productos de investigación in vitro
Tenga en cuenta que todos los artículos e información de productos presentados en BenchChem están destinados únicamente con fines informativos. Los productos disponibles para la compra en BenchChem están diseñados específicamente para estudios in vitro, que se realizan fuera de organismos vivos. Los estudios in vitro, derivados del término latino "in vidrio", involucran experimentos realizados en entornos de laboratorio controlados utilizando células o tejidos. Es importante tener en cuenta que estos productos no se clasifican como medicamentos y no han recibido la aprobación de la FDA para la prevención, tratamiento o cura de ninguna condición médica, dolencia o enfermedad. Debemos enfatizar que cualquier forma de introducción corporal de estos productos en humanos o animales está estrictamente prohibida por ley. Es esencial adherirse a estas pautas para garantizar el cumplimiento de los estándares legales y éticos en la investigación y experimentación.