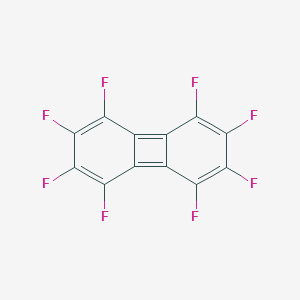
Octafluorobiphenylene
Descripción general
Descripción
Octafluorobiphenylene (OFBP) is a synthetic compound that has gained significant attention in the field of materials science and chemistry due to its unique properties. OFBP is a rigid, planar molecule consisting of two benzene rings connected by a double bond. The molecule is highly fluorinated, making it hydrophobic and resistant to degradation. OFBP has been studied for its potential applications in electronic devices, as well as in the synthesis of novel materials.
Aplicaciones Científicas De Investigación
Crystal and Molecular Structure : Octafluorobiphenylene has been synthesized and studied for its crystal and molecular structure. The crystal used in the study was grown from hexane, and the structure was analyzed using diffraction studies, revealing detailed information about its molecular arrangement (J. B. Jones et al., 1986).
Synthesis Methods : Different methods have been developed for synthesizing this compound. For example, it has been prepared by the pyrolysis of tetrafluorophthalic anhydride and by reacting the biphenylene with sodium methoxide or potassium hydroxide followed by diazomethane (D. Gardner et al., 1969).
Polymer Applications : this compound has been used in the creation of step-growth polymers. For instance, sodium indenide and decafluorobiphenyl react to afford a polymer with indene connected by this compound units. Such polymers have potential applications in various fields including materials science (P. A. Deck & Carrie R. Maiorana, 2001).
Electronic Applications : The use of this compound in electronics, particularly in OLEDs (Organic Light Emitting Diodes), has been explored. Research has been conducted on the synthesis of π-conjugated polymers containing fluorinated arylene units via direct arylation using octafluorobiphenyl, showing potential for efficient OLED materials (Wei Lu et al., 2012).
Coatings and Medical Devices : this compound derivatives, such as parylene AF4, have been developed for use as coatings in various applications, including medical devices and electronics. The synthesis and modification of these compounds have been a subject of research, highlighting their potential in diverse industrial applications (Craig Hicks et al., 2014).
Chemical Vapor Deposition : Research has also been conducted on the synthesis of precursors for chemical vapor deposition of parylene AF-4, a derivative of this compound. This includes the development of alternative precursors with improved reaction parameters and solvents (Daniel Kast et al., 2021).
Mecanismo De Acción
Target of Action
Octafluorobiphenylene is primarily used in the synthesis of conjugated polymers . Its primary targets are therefore the reactants and catalysts involved in these polymerization reactions .
Mode of Action
This compound interacts with its targets through a Pd-catalyzed cross-dehydrogenative-coupling reaction . This reaction involves the coupling of this compound with thiophene analogues, facilitated by a palladium catalyst .
Biochemical Pathways
The biochemical pathways affected by this compound are primarily related to the synthesis of conjugated polymers . The compound’s mode of action leads to the formation of an alternating π-conjugated polymer .
Pharmacokinetics
Its solubility, stability, and reactivity in various solvents and under different conditions are crucial for its use in polymer synthesis .
Result of Action
The result of this compound’s action is the formation of conjugated polymers with specific properties . These polymers have been evaluated as emitting materials for organic light-emitting diodes .
Propiedades
IUPAC Name |
1,2,3,4,5,6,7,8-octafluorobiphenylene | |
---|---|---|
Source | PubChem | |
URL | https://pubchem.ncbi.nlm.nih.gov | |
Description | Data deposited in or computed by PubChem | |
InChI |
InChI=1S/C12F8/c13-5-1-2(6(14)10(18)9(5)17)4-3(1)7(15)11(19)12(20)8(4)16 | |
Source | PubChem | |
URL | https://pubchem.ncbi.nlm.nih.gov | |
Description | Data deposited in or computed by PubChem | |
InChI Key |
CQKQSUKECBDFHR-UHFFFAOYSA-N | |
Source | PubChem | |
URL | https://pubchem.ncbi.nlm.nih.gov | |
Description | Data deposited in or computed by PubChem | |
Canonical SMILES |
C12=C(C3=C1C(=C(C(=C3F)F)F)F)C(=C(C(=C2F)F)F)F | |
Source | PubChem | |
URL | https://pubchem.ncbi.nlm.nih.gov | |
Description | Data deposited in or computed by PubChem | |
Molecular Formula |
C12F8 | |
Source | PubChem | |
URL | https://pubchem.ncbi.nlm.nih.gov | |
Description | Data deposited in or computed by PubChem | |
DSSTOX Substance ID |
DTXSID20379720 | |
Record name | Octafluorobiphenylene | |
Source | EPA DSSTox | |
URL | https://comptox.epa.gov/dashboard/DTXSID20379720 | |
Description | DSSTox provides a high quality public chemistry resource for supporting improved predictive toxicology. | |
Molecular Weight |
296.11 g/mol | |
Source | PubChem | |
URL | https://pubchem.ncbi.nlm.nih.gov | |
Description | Data deposited in or computed by PubChem | |
CAS RN |
13628-92-7 | |
Record name | Octafluorobiphenylene | |
Source | EPA DSSTox | |
URL | https://comptox.epa.gov/dashboard/DTXSID20379720 | |
Description | DSSTox provides a high quality public chemistry resource for supporting improved predictive toxicology. | |
Retrosynthesis Analysis
AI-Powered Synthesis Planning: Our tool employs the Template_relevance Pistachio, Template_relevance Bkms_metabolic, Template_relevance Pistachio_ringbreaker, Template_relevance Reaxys, Template_relevance Reaxys_biocatalysis model, leveraging a vast database of chemical reactions to predict feasible synthetic routes.
One-Step Synthesis Focus: Specifically designed for one-step synthesis, it provides concise and direct routes for your target compounds, streamlining the synthesis process.
Accurate Predictions: Utilizing the extensive PISTACHIO, BKMS_METABOLIC, PISTACHIO_RINGBREAKER, REAXYS, REAXYS_BIOCATALYSIS database, our tool offers high-accuracy predictions, reflecting the latest in chemical research and data.
Strategy Settings
Precursor scoring | Relevance Heuristic |
---|---|
Min. plausibility | 0.01 |
Model | Template_relevance |
Template Set | Pistachio/Bkms_metabolic/Pistachio_ringbreaker/Reaxys/Reaxys_biocatalysis |
Top-N result to add to graph | 6 |
Feasible Synthetic Routes
Q & A
A: Octafluorobiphenylene features a planar biphenylene core, where two benzene rings are fused together with a four-membered ring, and all eight hydrogen atoms are replaced by fluorine atoms []. This structure is confirmed by X-ray crystallography [, ]. The 19F NMR spectrum of this compound is particularly interesting, as it provides insights into the electronic environment of the fluorine atoms and confirms the molecule's symmetry [].
A: this compound is explored as a building block for high-performance polymers. For instance, when incorporated into poly(arylene ether)s, it imparts high glass transition temperatures (above 279°C) and excellent thermal stability (decomposition temperatures exceeding 472°C) []. These properties make them attractive for applications requiring heat resistance, such as in advanced materials for electronics or aerospace.
A: Researchers have utilized this compound in the synthesis of polymers containing both azobenzene and azomethine units []. These polymers exhibit interesting photo-responsive behavior, undergoing changes in birefringence when exposed to UV and visible light. This property makes them potentially useful for applications like optical storage and controlling liquid crystal orientation [].
A: this compound exhibits reactivity with nucleophiles. For example, it undergoes nucleophilic substitution with methoxide ion, first at the 3-position and then at the 7-position []. The regioselectivity of these substitutions is influenced by the electronic effects of the fluorine atoms and the inherent reactivity of the different positions on the biphenylene core.
A: Yes, this compound serves as a valuable starting material for preparing other fluorinated compounds. It can be converted to heptafluoro-1-methoxybiphenylene and heptafluoro-2-methoxybiphenylene through a series of reactions involving potassium hydroxide and diazomethane []. This example highlights the potential of this compound as a versatile precursor in fluorine chemistry.
A: Researchers have successfully employed various polymerization techniques with this compound. One approach involves a palladium-catalyzed cross-dehydrogenative-coupling reaction with thiophene analogues to create donor-acceptor type π-conjugated polymers []. Another strategy utilizes decafluorobiphenyl and sodium indenide to generate a step-growth polymer, where indene units are linked by 4,4′-octafluorobiphenylene units [].
A: The rigid and planar structure of this compound significantly influences the properties of the polymers it forms. For example, in poly(azomethine)s, the presence of this compound contributes to high thermal stability and affects the polymer's optical properties []. The electron-withdrawing nature of the fluorine atoms also plays a role in dictating the polymer's overall electronic properties.
A: Computational methods are invaluable tools for studying this compound. Researchers utilize quantum chemical calculations to explore the singlet-triplet energy gap and diradical character of Chichibabin's hydrocarbon containing an this compound spacer []. These calculations help to explain the observed reactivity and properties of these systems.
Descargo de responsabilidad e información sobre productos de investigación in vitro
Tenga en cuenta que todos los artículos e información de productos presentados en BenchChem están destinados únicamente con fines informativos. Los productos disponibles para la compra en BenchChem están diseñados específicamente para estudios in vitro, que se realizan fuera de organismos vivos. Los estudios in vitro, derivados del término latino "in vidrio", involucran experimentos realizados en entornos de laboratorio controlados utilizando células o tejidos. Es importante tener en cuenta que estos productos no se clasifican como medicamentos y no han recibido la aprobación de la FDA para la prevención, tratamiento o cura de ninguna condición médica, dolencia o enfermedad. Debemos enfatizar que cualquier forma de introducción corporal de estos productos en humanos o animales está estrictamente prohibida por ley. Es esencial adherirse a estas pautas para garantizar el cumplimiento de los estándares legales y éticos en la investigación y experimentación.