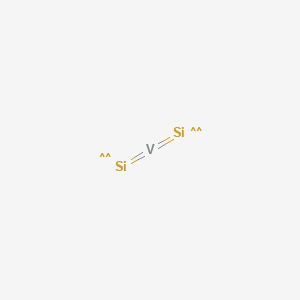
Silicuro de vanadio (VSi2)
Descripción general
Descripción
Vanadium silicide is an intermetallic compound composed of vanadium and silicon. It is known for its high melting point, hardness, and excellent thermal stability. Vanadium silicide is primarily used in high-temperature applications and as a material for thin-film coatings in microelectronics.
Aplicaciones Científicas De Investigación
Vanadium silicide has a wide range of scientific research applications due to its unique properties. In chemistry, it is used as a catalyst for various reactions. In microelectronics, vanadium silicide is used as a material for thin-film coatings and interconnects due to its excellent electrical conductivity and thermal stability . In the field of materials science, vanadium silicide is studied for its potential use in high-temperature structural materials .
Mecanismo De Acción
Target of Action
Vanadium silicide (VSi2) primarily targets silicon (Si) surfaces, particularly the Si(111) surface . The interaction between vanadium and silicon forms a two-dimensional network of VSi2 clusters .
Mode of Action
The mode of action of VSi2 involves the deposition of vanadium on a silicon surface followed by annealing . This process leads to the formation of VSi2 clusters. The shape of these clusters can vary depending on the amount of vanadium deposited. For instance, the deposition of 8 monolayers of vanadium on Si(111) and subsequent annealing at 1200 K leads to the formation of large elongated VSi2 clusters . This is in contrast to the deposition of submonolayer amounts of V where the VSi2 islands have a compact shape .
Biochemical Pathways
The biochemical pathways affected by VSi2 are primarily related to the growth and formation of vanadium silicide clusters on silicon surfaces . The shape transition of these clusters can be explained by a competition between edge formation and strain relaxation energy terms .
Pharmacokinetics
The diffusion of vanadium is a critical factor in the formation of vsi2 clusters .
Result of Action
The result of the action of VSi2 is the formation of a two-dimensional network of VSi2 clusters on silicon surfaces . These clusters are aligned along the three high symmetry directions of the Si(111) surface and are surrounded by a denuded zone .
Action Environment
The action of VSi2 is influenced by environmental factors such as temperature and the amount of vanadium deposited on the silicon surface . For instance, the shape of the VSi2 clusters changes with the amount of vanadium deposited, and the annealing temperature affects the formation of these clusters .
Métodos De Preparación
Synthetic Routes and Reaction Conditions: Vanadium silicide can be synthesized through various methods, including solid-state reactions, chemical vapor deposition, and sputtering techniques. One common method involves the reaction of vanadium and silicon at high temperatures (600 to 1000°C) to form vanadium silicide . Another method involves the deposition of vanadium on silicon substrates followed by annealing at elevated temperatures to promote the formation of vanadium silicide clusters .
Industrial Production Methods: In industrial settings, vanadium silicide is often produced using chemical vapor deposition or sputtering techniques. These methods allow for precise control over the composition and thickness of the vanadium silicide films, making them suitable for use in microelectronics and other high-tech applications .
Análisis De Reacciones Químicas
Types of Reactions: Vanadium silicide undergoes various chemical reactions, including oxidation, reduction, and substitution reactions. For example, when exposed to oxygen at high temperatures, vanadium silicide can form vanadium oxides and silicon dioxide .
Common Reagents and Conditions: Common reagents used in the reactions of vanadium silicide include oxygen, hydrogen, and halogens. The reactions typically occur at elevated temperatures, often above 600°C .
Major Products Formed: The major products formed from the reactions of vanadium silicide include vanadium oxides, silicon dioxide, and other vanadium-silicon compounds .
Comparación Con Compuestos Similares
- Vanadium disilicide (V3Si)
- Vanadium pentasilicide (V5Si3)
- Titanium silicide (TiSi2)
- Molybdenum silicide (MoSi2)
Comparison: Vanadium silicide is unique among these compounds due to its specific phase stability and formation conditions. Unlike vanadium disilicide, which forms at lower silicon concentrations, vanadium silicide requires higher silicon content and higher temperatures for its formation . Compared to titanium silicide and molybdenum silicide, vanadium silicide offers a different balance of electrical conductivity and thermal stability, making it suitable for specific high-temperature applications .
Actividad Biológica
Vanadium silicide (VSi2) is a compound that has garnered attention in both materials science and biomedicine due to its unique properties and potential biological activities. This article explores the biological implications of VSi2, focusing on its mechanisms of action, effects on cellular processes, and potential therapeutic applications.
Overview of Vanadium Silicide
Vanadium silicide is a silicide of vanadium, primarily known for its applications in metallurgy and electronics due to its high melting point and good electrical conductivity. However, recent studies have indicated that VSi2 may also exhibit notable biological activities, particularly in relation to oxidative stress and cellular metabolism.
Mechanisms of Biological Activity
-
Oxidative Stress Modulation :
- Vanadium compounds, including VSi2, are known to influence oxidative stress pathways. They can induce lipid peroxidation (LPO), a process detrimental to cellular membranes that leads to the formation of reactive oxygen species (ROS) . The modulation of oxidative stress is critical in various disease states, including cancer and diabetes.
- Enzymatic Regulation :
- Cellular Interaction :
Table 1: Summary of Biological Effects of VSi2
Detailed Research Findings
- Lipid Peroxidation and Oxidative Stress : A comprehensive review highlighted how vanadium species can induce LPO through oxidative mechanisms, affecting mitochondrial function and cellular integrity . The study emphasized the need for further speciation studies to understand the diverse effects of vanadium compounds under physiological conditions.
- Effects on Immune Cells : In vitro experiments demonstrated that VSi2 influences neutrophil behavior, leading to increased production of inflammatory mediators such as superoxide anions. This suggests a dual role where VSi2 could potentially enhance immune responses while also contributing to oxidative damage if not regulated properly .
- Therapeutic Potential : Given its biological activities, VSi2 may hold promise as a therapeutic agent in conditions characterized by oxidative stress and metabolic dysregulation. Its ability to modulate enzymatic pathways could be leveraged for developing treatments for diabetes and other metabolic disorders .
Propiedades
InChI |
InChI=1S/2Si.V | |
---|---|---|
Source | PubChem | |
URL | https://pubchem.ncbi.nlm.nih.gov | |
Description | Data deposited in or computed by PubChem | |
InChI Key |
LUWOVYQXZRKECH-UHFFFAOYSA-N | |
Source | PubChem | |
URL | https://pubchem.ncbi.nlm.nih.gov | |
Description | Data deposited in or computed by PubChem | |
Canonical SMILES |
[Si]=[V]=[Si] | |
Source | PubChem | |
URL | https://pubchem.ncbi.nlm.nih.gov | |
Description | Data deposited in or computed by PubChem | |
Molecular Formula |
Si2V | |
Source | PubChem | |
URL | https://pubchem.ncbi.nlm.nih.gov | |
Description | Data deposited in or computed by PubChem | |
Molecular Weight |
107.11 g/mol | |
Source | PubChem | |
URL | https://pubchem.ncbi.nlm.nih.gov | |
Description | Data deposited in or computed by PubChem | |
CAS No. |
12039-87-1 | |
Record name | Vanadium silicide (VSi2) | |
Source | CAS Common Chemistry | |
URL | https://commonchemistry.cas.org/detail?cas_rn=12039-87-1 | |
Description | CAS Common Chemistry is an open community resource for accessing chemical information. Nearly 500,000 chemical substances from CAS REGISTRY cover areas of community interest, including common and frequently regulated chemicals, and those relevant to high school and undergraduate chemistry classes. This chemical information, curated by our expert scientists, is provided in alignment with our mission as a division of the American Chemical Society. | |
Explanation | The data from CAS Common Chemistry is provided under a CC-BY-NC 4.0 license, unless otherwise stated. | |
Record name | Vanadium silicide (VSi2) | |
Source | EPA Chemicals under the TSCA | |
URL | https://www.epa.gov/chemicals-under-tsca | |
Description | EPA Chemicals under the Toxic Substances Control Act (TSCA) collection contains information on chemicals and their regulations under TSCA, including non-confidential content from the TSCA Chemical Substance Inventory and Chemical Data Reporting. | |
Record name | Vanadium disilicide | |
Source | European Chemicals Agency (ECHA) | |
URL | https://echa.europa.eu/substance-information/-/substanceinfo/100.031.722 | |
Description | The European Chemicals Agency (ECHA) is an agency of the European Union which is the driving force among regulatory authorities in implementing the EU's groundbreaking chemicals legislation for the benefit of human health and the environment as well as for innovation and competitiveness. | |
Explanation | Use of the information, documents and data from the ECHA website is subject to the terms and conditions of this Legal Notice, and subject to other binding limitations provided for under applicable law, the information, documents and data made available on the ECHA website may be reproduced, distributed and/or used, totally or in part, for non-commercial purposes provided that ECHA is acknowledged as the source: "Source: European Chemicals Agency, http://echa.europa.eu/". Such acknowledgement must be included in each copy of the material. ECHA permits and encourages organisations and individuals to create links to the ECHA website under the following cumulative conditions: Links can only be made to webpages that provide a link to the Legal Notice page. | |
Q1: What is the nature of bonding in VSi2 and how is charge transfer involved?
A2: Studies using γ-ray Compton scattering techniques indicate that charge transfer occurs from silicon to vanadium atoms within VSi2. [] This charge transfer phenomenon suggests a degree of ionic character in the bonding alongside the expected covalent interactions between vanadium and silicon atoms. While the exact nature of this bonding requires further theoretical exploration, a superposition model, incorporating both ionic and covalent contributions, might be suitable for describing the bonding in VSi2. []
Descargo de responsabilidad e información sobre productos de investigación in vitro
Tenga en cuenta que todos los artículos e información de productos presentados en BenchChem están destinados únicamente con fines informativos. Los productos disponibles para la compra en BenchChem están diseñados específicamente para estudios in vitro, que se realizan fuera de organismos vivos. Los estudios in vitro, derivados del término latino "in vidrio", involucran experimentos realizados en entornos de laboratorio controlados utilizando células o tejidos. Es importante tener en cuenta que estos productos no se clasifican como medicamentos y no han recibido la aprobación de la FDA para la prevención, tratamiento o cura de ninguna condición médica, dolencia o enfermedad. Debemos enfatizar que cualquier forma de introducción corporal de estos productos en humanos o animales está estrictamente prohibida por ley. Es esencial adherirse a estas pautas para garantizar el cumplimiento de los estándares legales y éticos en la investigación y experimentación.