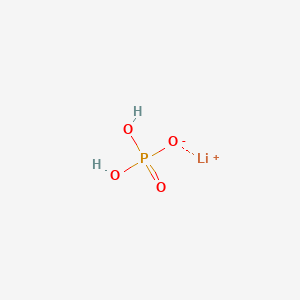
Lithium dihydrogen phosphate
Descripción general
Descripción
Lithium Dihydrogen Phosphate (LiH2PO4) is a chemical compound of lithium. It appears as a white powder and is mainly used for the preparation of lithium iron phosphate cathode material for lithium-ion batteries .
Synthesis Analysis
LiH2PO4 is usually prepared by a stoichiometric reaction of Li2CO3 and 85% orthophosphoric acid . Another method involves the reaction of Li3PO4 with H3PO4 to form LiH2PO4 . It can also be formed by the reaction of LiOH with H3PO4 .Chemical Reactions Analysis
LiH2PO4 reacts with water to produce lithium hydroxide and hydrogen phosphate . It is also used in the preparation method of lithium hydroxide from lithium-containing low-magnesium brine in a lithium phosphate manner .Physical And Chemical Properties Analysis
LiH2PO4 is a white crystalline powder . It has a molecular weight of 103.93 . It is slightly soluble in water . The melting point is greater than 100℃ . It is stable and incompatible with strong oxidizing agents .Aplicaciones Científicas De Investigación
Ionic Conductor
Lithium dihydrogen phosphate (LDP) is studied as an ionic conductor . The electronic surroundings of phosphorus and lithium atoms in LDP have been studied by single-crystal nuclear magnetic resonance (NMR) spectroscopy . This conductivity is predominantly attributed to fast proton movements in the crystal lattice of LDP . This makes it a promising material for fuel cell or rechargeable battery applications .
Cathode Material for Lithium-Ion Batteries
LDP is used as a precursor for LiMX2O7 (M = Fe, V; x =P, As) cathode materials . Lithium iron phosphate (LiFePO4) based material is one of the most prospective candidates as a cathode material in lithium-ion batteries because of its lower cost, safer, and environmental benignity .
AC and DC Conductivity Study
The electrical properties of LDP were studied using complex impedance spectroscopy as a function of frequency at various temperatures . The frequency dependence of the conductivity is interpreted in terms of Jonscher’s law . The transport is through ion hopping mechanism dominated by the motion of the proton in the structure of the investigated material .
Structural and Electronic Characterization
LDP is widely available, making it a promising material for precise structural and electronic characterization . As a well-established analytical technique, Nuclear Magnetic Resonance (NMR) spectroscopy is capable of providing information on structure and dynamics of solids .
Fuel Cell Applications
Due to its high conductivity, LDP is considered a promising compound for a membrane in medium-temperature fuel cells .
Electrochemical Devices
Alkali metal dihydrogen phosphate type compounds, including LDP, have important applications such as fuel cell, proton batteries, and electrochemical devices .
Mecanismo De Acción
Target of Action
Lithium dihydrogen phosphate (LiH2PO4) is an important material in battery technology and catalysis . Its primary targets are the biochemical pathways and processes involved in these applications.
Mode of Action
LiH2PO4 interacts with its targets through a process known as nucleation, which can be described by a power law resembling mass action kinetics . The constructed reaction kinetic model includes consecutive reversible complex formation preceding the heterogeneous reaction .
Biochemical Pathways
LiH2PO4 affects several biochemical pathways. It competes with other cations, especially sodium and magnesium, for macromolecular binding sites . This competition modulates the biochemical phenotype .
Pharmacokinetics
The pharmacokinetics of LiH2PO4 involve its absorption, distribution, metabolism, and excretion (ADME). The compound is crystallized in the orthorhombic system . Its electrical properties have been studied using complex impedance spectroscopy, revealing that the transport is through an ion hopping mechanism dominated by the motion of the proton in the structure of the investigated material .
Result of Action
The action of LiH2PO4 results in changes at the molecular and cellular levels. Its interaction with its targets leads to changes in the conductivity of the material , which is crucial for its applications in battery technology and catalysis .
Action Environment
The action, efficacy, and stability of LiH2PO4 are influenced by environmental factors. For instance, its stability is affected by temperature and humidity . It has been shown that pure LiH2PO4, in the presence of sufficient humidity, is stable up to 360 °C . Furthermore, the compound’s conductivity is influenced by the frequency and temperature .
Safety and Hazards
Direcciones Futuras
Propiedades
IUPAC Name |
lithium;dihydrogen phosphate | |
---|---|---|
Source | PubChem | |
URL | https://pubchem.ncbi.nlm.nih.gov | |
Description | Data deposited in or computed by PubChem | |
InChI |
InChI=1S/Li.H3O4P/c;1-5(2,3)4/h;(H3,1,2,3,4)/q+1;/p-1 | |
Source | PubChem | |
URL | https://pubchem.ncbi.nlm.nih.gov | |
Description | Data deposited in or computed by PubChem | |
InChI Key |
SNKMVYBWZDHJHE-UHFFFAOYSA-M | |
Source | PubChem | |
URL | https://pubchem.ncbi.nlm.nih.gov | |
Description | Data deposited in or computed by PubChem | |
Canonical SMILES |
[Li+].OP(=O)(O)[O-] | |
Source | PubChem | |
URL | https://pubchem.ncbi.nlm.nih.gov | |
Description | Data deposited in or computed by PubChem | |
Molecular Formula |
LiH2PO4, H2LiO4P | |
Record name | monolithium phosphate | |
Source | Wikipedia | |
URL | https://en.wikipedia.org/wiki/Dictionary_of_chemical_formulas | |
Description | Chemical information link to Wikipedia. | |
Source | PubChem | |
URL | https://pubchem.ncbi.nlm.nih.gov | |
Description | Data deposited in or computed by PubChem | |
DSSTOX Substance ID |
DTXSID40884609 | |
Record name | Phosphoric acid, lithium salt (1:1) | |
Source | EPA DSSTox | |
URL | https://comptox.epa.gov/dashboard/DTXSID40884609 | |
Description | DSSTox provides a high quality public chemistry resource for supporting improved predictive toxicology. | |
Molecular Weight |
104.0 g/mol | |
Source | PubChem | |
URL | https://pubchem.ncbi.nlm.nih.gov | |
Description | Data deposited in or computed by PubChem | |
Product Name |
Lithium dihydrogen phosphate | |
CAS RN |
13453-80-0 | |
Record name | Phosphoric acid, lithium salt (1:1) | |
Source | ChemIDplus | |
URL | https://pubchem.ncbi.nlm.nih.gov/substance/?source=chemidplus&sourceid=0013453800 | |
Description | ChemIDplus is a free, web search system that provides access to the structure and nomenclature authority files used for the identification of chemical substances cited in National Library of Medicine (NLM) databases, including the TOXNET system. | |
Record name | Phosphoric acid, lithium salt (1:1) | |
Source | EPA Chemicals under the TSCA | |
URL | https://www.epa.gov/chemicals-under-tsca | |
Description | EPA Chemicals under the Toxic Substances Control Act (TSCA) collection contains information on chemicals and their regulations under TSCA, including non-confidential content from the TSCA Chemical Substance Inventory and Chemical Data Reporting. | |
Record name | Phosphoric acid, lithium salt (1:1) | |
Source | EPA DSSTox | |
URL | https://comptox.epa.gov/dashboard/DTXSID40884609 | |
Description | DSSTox provides a high quality public chemistry resource for supporting improved predictive toxicology. | |
Record name | Lithium dihydrogenorthophosphate | |
Source | European Chemicals Agency (ECHA) | |
URL | https://echa.europa.eu/substance-information/-/substanceinfo/100.033.289 | |
Description | The European Chemicals Agency (ECHA) is an agency of the European Union which is the driving force among regulatory authorities in implementing the EU's groundbreaking chemicals legislation for the benefit of human health and the environment as well as for innovation and competitiveness. | |
Explanation | Use of the information, documents and data from the ECHA website is subject to the terms and conditions of this Legal Notice, and subject to other binding limitations provided for under applicable law, the information, documents and data made available on the ECHA website may be reproduced, distributed and/or used, totally or in part, for non-commercial purposes provided that ECHA is acknowledged as the source: "Source: European Chemicals Agency, http://echa.europa.eu/". Such acknowledgement must be included in each copy of the material. ECHA permits and encourages organisations and individuals to create links to the ECHA website under the following cumulative conditions: Links can only be made to webpages that provide a link to the Legal Notice page. | |
Retrosynthesis Analysis
AI-Powered Synthesis Planning: Our tool employs the Template_relevance Pistachio, Template_relevance Bkms_metabolic, Template_relevance Pistachio_ringbreaker, Template_relevance Reaxys, Template_relevance Reaxys_biocatalysis model, leveraging a vast database of chemical reactions to predict feasible synthetic routes.
One-Step Synthesis Focus: Specifically designed for one-step synthesis, it provides concise and direct routes for your target compounds, streamlining the synthesis process.
Accurate Predictions: Utilizing the extensive PISTACHIO, BKMS_METABOLIC, PISTACHIO_RINGBREAKER, REAXYS, REAXYS_BIOCATALYSIS database, our tool offers high-accuracy predictions, reflecting the latest in chemical research and data.
Strategy Settings
Precursor scoring | Relevance Heuristic |
---|---|
Min. plausibility | 0.01 |
Model | Template_relevance |
Template Set | Pistachio/Bkms_metabolic/Pistachio_ringbreaker/Reaxys/Reaxys_biocatalysis |
Top-N result to add to graph | 6 |
Feasible Synthetic Routes
Descargo de responsabilidad e información sobre productos de investigación in vitro
Tenga en cuenta que todos los artículos e información de productos presentados en BenchChem están destinados únicamente con fines informativos. Los productos disponibles para la compra en BenchChem están diseñados específicamente para estudios in vitro, que se realizan fuera de organismos vivos. Los estudios in vitro, derivados del término latino "in vidrio", involucran experimentos realizados en entornos de laboratorio controlados utilizando células o tejidos. Es importante tener en cuenta que estos productos no se clasifican como medicamentos y no han recibido la aprobación de la FDA para la prevención, tratamiento o cura de ninguna condición médica, dolencia o enfermedad. Debemos enfatizar que cualquier forma de introducción corporal de estos productos en humanos o animales está estrictamente prohibida por ley. Es esencial adherirse a estas pautas para garantizar el cumplimiento de los estándares legales y éticos en la investigación y experimentación.