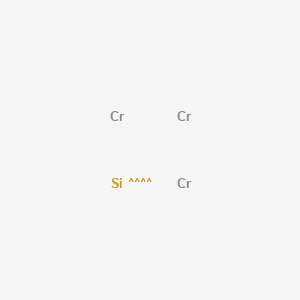
Chromium silicide (Cr3Si)
Descripción general
Descripción
Chromium silicide (Cr3Si) is an intermetallic compound composed of chromium and silicon. It crystallizes in the cubic Pm-3n space group and exhibits a three-dimensional structure where chromium atoms are bonded in a 6-coordinate geometry to two equivalent chromium atoms and four equivalent silicon atoms . Chromium silicide is known for its high melting point, excellent mechanical properties, and outstanding resistance to high-temperature oxidation .
Métodos De Preparación
Synthetic Routes and Reaction Conditions: Chromium silicide can be synthesized through various methods. One common approach is the solid-state reaction of chromium sesquioxide, silicon powder, and metallic lithium in an autoclave at 600°C for 10 hours . This method yields pure cubic chromium silicide hollow particles. Another method involves self-propagating high-temperature synthesis, where a chromium-silicon alloy is fabricated for coating metal surfaces .
Industrial Production Methods: In industrial settings, chromium silicide is often produced using high-temperature furnaces and controlled atmospheres to ensure the purity and quality of the final product. The use of water-cooled copper mold laser furnaces has also been reported for the preparation of chromium-silicon alloys .
Análisis De Reacciones Químicas
Types of Reactions: Chromium silicide undergoes various chemical reactions, including oxidation and reduction. For instance, it begins to oxidize in air at 650°C, forming a protective layer that enhances its thermal oxidation stability up to 1000°C .
Common Reagents and Conditions: The oxidation of chromium silicide typically involves exposure to air or oxygen at elevated temperatures. The reduction reactions may involve the use of reducing agents such as hydrogen or carbon monoxide under controlled conditions.
Major Products Formed: The primary product formed during the oxidation of chromium silicide is a protective oxide layer that prevents further oxidation. This layer contributes to the compound’s high thermal stability and resistance to oxidation .
Aplicaciones Científicas De Investigación
High-Temperature Coatings
In the electronics sector, chromium silicide is employed for high-temperature-resistant coatings. Its thermal stability makes it ideal for components exposed to elevated temperatures, such as semiconductor devices and microelectronics .
Schottky Contacts
Chromium silicide has been investigated for its role in Schottky contacts in semiconductor devices. Studies utilizing X-ray Absorption Fine Structure spectroscopy have shown that chromium diffusion into amorphous silicon can lead to the formation of various chromium phases, which are crucial for optimizing interface properties in electronic applications .
Thermoelectric Devices
Chromium silicide is also explored for its potential use in thermoelectric devices. The compound's ability to maintain structural integrity at high temperatures while exhibiting favorable electrical properties makes it a candidate for energy conversion applications .
Case Studies
Case Study 1: Aerospace Applications
A study on Cr-Si alloys demonstrated that adding chromium silicide significantly improved oxidation resistance and mechanical performance under high-temperature conditions typical in aerospace environments. The results indicated a marked increase in service life compared to traditional materials used in similar applications .
Case Study 2: Semiconductor Interfaces
Research involving X-ray Absorption Fine Structure spectroscopy revealed that annealing Cr/a-Si interfaces at varying temperatures led to phase transformations critical for enhancing Schottky contact performance. The findings underscore the importance of chromium silicide in optimizing electronic device efficiency .
Mecanismo De Acción
Chromium silicide can be compared with other similar compounds, such as chromium disilicide (CrSi2) and chromium pentasilicide (Cr5Si3). These compounds also exhibit high melting points and excellent mechanical properties but differ in their crystal structures and specific applications .
Comparación Con Compuestos Similares
- Chromium disilicide (CrSi2)
- Chromium pentasilicide (Cr5Si3)
- Chromium silicon (CrSi)
Chromium silicide (Cr3Si) stands out due to its unique cubic crystal structure and the ability to form protective oxide layers that enhance its thermal stability and resistance to oxidation .
Actividad Biológica
Chromium silicide (Cr₃Si) is a compound of significant interest due to its unique properties and potential applications in various fields, including electronics, materials science, and biology. This article delves into the biological activity of Cr₃Si, summarizing research findings, synthesizing data, and presenting case studies that highlight its interactions within biological systems.
Overview of Chromium Silicide
Chromium silicide is a transition metal silicide known for its high melting point, good thermal stability, and electrical conductivity. It exists in several phases, with Cr₃Si being one of the most studied due to its cubic crystal structure and favorable mechanical properties. Understanding its biological activity is crucial, especially considering its use in various industrial applications.
Synthesis and Characterization
Cr₃Si can be synthesized through various methods, including solid-state reactions and pack cementation processes. For instance, a study reported the synthesis of pure cubic Cr₃Si hollow particles using chromium sesquioxide and silicon powder at elevated temperatures (600 °C) in an autoclave . Characterization techniques such as X-ray diffraction (XRD) and scanning electron microscopy (SEM) have been employed to confirm the structural integrity and morphology of the synthesized particles.
Toxicity Profile
Research indicates that Cr₃Si may pose certain health risks. Exposure to chromium compounds has been linked to respiratory issues, skin irritation, and potential carcinogenic effects. Specifically, inhalation of chromium silicide dust can lead to chronic respiratory conditions due to lung fibrosis . The compound can also cause skin and eye irritation upon direct contact.
Cellular Interactions
Studies have explored the cellular interactions of Cr₃Si. One investigation focused on the cytotoxic effects of Cr₃Si on human lung fibroblast cells. The results demonstrated that exposure to varying concentrations of Cr₃Si resulted in dose-dependent cytotoxicity, leading to increased cell death rates at higher concentrations . This suggests that while Cr₃Si has potential applications in biocompatible materials, careful consideration must be given to its cytotoxic effects.
Case Studies
- In Vivo Studies : A notable study investigated the effects of Cr₃Si nanoparticles on murine models. The findings revealed that these nanoparticles could induce inflammatory responses when administered intranasally. Histological examinations showed significant lung inflammation and damage, indicating that while Cr₃Si has promising properties for certain applications, its biological safety profile requires further scrutiny .
- Biocompatibility Assessments : Another case study assessed the biocompatibility of Cr₃Si coatings used in orthopedic implants. The study found that while the coatings exhibited good mechanical properties and corrosion resistance, they also triggered mild inflammatory responses in surrounding tissues when implanted in animal models . This highlights the need for thorough biocompatibility testing before clinical applications.
Data Summary
The following table summarizes key findings related to the biological activity of Cr₃Si:
Propiedades
InChI |
InChI=1S/3Cr.Si | |
---|---|---|
Source | PubChem | |
URL | https://pubchem.ncbi.nlm.nih.gov | |
Description | Data deposited in or computed by PubChem | |
InChI Key |
ITRGGKFLQODYDH-UHFFFAOYSA-N | |
Source | PubChem | |
URL | https://pubchem.ncbi.nlm.nih.gov | |
Description | Data deposited in or computed by PubChem | |
Canonical SMILES |
[Si].[Cr].[Cr].[Cr] | |
Source | PubChem | |
URL | https://pubchem.ncbi.nlm.nih.gov | |
Description | Data deposited in or computed by PubChem | |
Molecular Formula |
Cr3Si | |
Source | PubChem | |
URL | https://pubchem.ncbi.nlm.nih.gov | |
Description | Data deposited in or computed by PubChem | |
Molecular Weight |
184.07 g/mol | |
Source | PubChem | |
URL | https://pubchem.ncbi.nlm.nih.gov | |
Description | Data deposited in or computed by PubChem | |
Physical Description |
Black odorless pieces and powder; Insoluble in water; [Materion Advanced Chemicals MSDS] | |
Record name | Trichromium silicide | |
Source | Haz-Map, Information on Hazardous Chemicals and Occupational Diseases | |
URL | https://haz-map.com/Agents/19895 | |
Description | Haz-Map® is an occupational health database designed for health and safety professionals and for consumers seeking information about the adverse effects of workplace exposures to chemical and biological agents. | |
Explanation | Copyright (c) 2022 Haz-Map(R). All rights reserved. Unless otherwise indicated, all materials from Haz-Map are copyrighted by Haz-Map(R). No part of these materials, either text or image may be used for any purpose other than for personal use. Therefore, reproduction, modification, storage in a retrieval system or retransmission, in any form or by any means, electronic, mechanical or otherwise, for reasons other than personal use, is strictly prohibited without prior written permission. | |
CAS No. |
12018-36-9 | |
Record name | Chromium silicide (Cr3Si) | |
Source | ChemIDplus | |
URL | https://pubchem.ncbi.nlm.nih.gov/substance/?source=chemidplus&sourceid=0012018369 | |
Description | ChemIDplus is a free, web search system that provides access to the structure and nomenclature authority files used for the identification of chemical substances cited in National Library of Medicine (NLM) databases, including the TOXNET system. | |
Record name | Chromium silicide (Cr3Si) | |
Source | EPA Chemicals under the TSCA | |
URL | https://www.epa.gov/chemicals-under-tsca | |
Description | EPA Chemicals under the Toxic Substances Control Act (TSCA) collection contains information on chemicals and their regulations under TSCA, including non-confidential content from the TSCA Chemical Substance Inventory and Chemical Data Reporting. | |
Record name | Trichromium silicide | |
Source | European Chemicals Agency (ECHA) | |
URL | https://echa.europa.eu/substance-information/-/substanceinfo/100.031.478 | |
Description | The European Chemicals Agency (ECHA) is an agency of the European Union which is the driving force among regulatory authorities in implementing the EU's groundbreaking chemicals legislation for the benefit of human health and the environment as well as for innovation and competitiveness. | |
Explanation | Use of the information, documents and data from the ECHA website is subject to the terms and conditions of this Legal Notice, and subject to other binding limitations provided for under applicable law, the information, documents and data made available on the ECHA website may be reproduced, distributed and/or used, totally or in part, for non-commercial purposes provided that ECHA is acknowledged as the source: "Source: European Chemicals Agency, http://echa.europa.eu/". Such acknowledgement must be included in each copy of the material. ECHA permits and encourages organisations and individuals to create links to the ECHA website under the following cumulative conditions: Links can only be made to webpages that provide a link to the Legal Notice page. | |
Descargo de responsabilidad e información sobre productos de investigación in vitro
Tenga en cuenta que todos los artículos e información de productos presentados en BenchChem están destinados únicamente con fines informativos. Los productos disponibles para la compra en BenchChem están diseñados específicamente para estudios in vitro, que se realizan fuera de organismos vivos. Los estudios in vitro, derivados del término latino "in vidrio", involucran experimentos realizados en entornos de laboratorio controlados utilizando células o tejidos. Es importante tener en cuenta que estos productos no se clasifican como medicamentos y no han recibido la aprobación de la FDA para la prevención, tratamiento o cura de ninguna condición médica, dolencia o enfermedad. Debemos enfatizar que cualquier forma de introducción corporal de estos productos en humanos o animales está estrictamente prohibida por ley. Es esencial adherirse a estas pautas para garantizar el cumplimiento de los estándares legales y éticos en la investigación y experimentación.