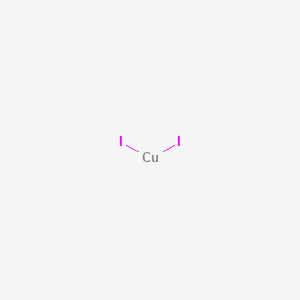
diiodocopper
Descripción general
Descripción
diiodocopper is an inorganic compound formed by copper in its +2 oxidation state and iodine. It is less stable compared to copper(I) iodide (CuI) and often decomposes to iodine and copper(I) iodide in the presence of moisture . This compound is notable for its unique properties and applications in various fields.
Métodos De Preparación
Copper iodide can be prepared in a laboratory by reacting iodine with copper. The reaction can be represented by the following equation: [ \text{Cu (s) + I}_2 \text{(s) → CuI (s)} ] Industrial production often involves the reaction of copper sulfate with potassium iodide. The copper iodide precipitates out of the solution and can then be collected . Another method involves reacting copper(II) sulfate and potassium iodide, which produces copper(II) iodide that immediately decomposes to iodine and copper(I) iodide .
Análisis De Reacciones Químicas
Copper iodide undergoes various types of reactions, including:
Oxidation: Copper iodide can be oxidized to form copper(II) iodide and iodine.
Reduction: It acts as a reducing agent, easily oxidizing to iodine.
Substitution: Copper iodide can catalyze the substitution reactions, such as the conversion of aryl halides to aryl iodides.
Common reagents and conditions used in these reactions include thiourea, alkyl bromides, and potassium carbonate in wet polyethylene glycol . Major products formed from these reactions include aryl sulfides and transition metal alkynyl complexes .
Aplicaciones Científicas De Investigación
Chemical Applications
Catalysis in Organic Synthesis
- Diiodocopper serves as a catalyst in several organic synthesis reactions. It is particularly effective in coupling reactions, where it facilitates the formation of carbon-carbon bonds. Its role in thioetherification reactions is also noteworthy, enabling the conversion of aryl halides to aryl iodides.
Redox Reactions
- The compound participates in redox reactions where it can be oxidized to form copper(II) iodide and iodine. This property makes it useful in various chemical transformations and as a reducing agent in organic synthesis.
Biological Applications
Antimicrobial Properties
- Research has highlighted the antimicrobial activity of copper iodide nanoparticles against various pathogens. Studies indicate that these nanoparticles can effectively inhibit microbial growth by disrupting cell membranes, making them suitable for biomedical applications .
Virucidal Activity Against SARS-CoV-2
- A significant application of this compound is its use in combating viral infections, particularly SARS-CoV-2. Copper iodide nanoparticles have been shown to rapidly inactivate the virus through mechanisms involving the generation of reactive oxygen species, which damage viral proteins and RNA. This property positions CuI as a promising material for antiviral coatings on high-touch surfaces and protective clothing .
Material Science Applications
High-Intensity Discharge Lamps
- This compound is utilized in the production of high-intensity discharge (HID) lamps, where it enhances color rendering and energy efficiency. Its ability to stabilize light emissions makes it valuable for improving lighting technologies.
Cloud Seeding
- In meteorological applications, this compound is explored for cloud seeding, where it acts as a nucleating agent to promote precipitation by providing surfaces for moisture condensation.
Case Studies and Research Findings
Mecanismo De Acción
Copper iodide exerts its effects through various mechanisms:
Catalysis: It acts as a catalyst in organic reactions by facilitating the formation of transition metal complexes.
Antimicrobial Activity: Copper iodide nanoparticles hinder microbial colonization by disrupting the cell membranes of pathogens.
Cloud Seeding: Copper iodide provides a nucleus for moisture in clouds to condense around, increasing precipitation.
Comparación Con Compuestos Similares
Copper iodide can be compared with other similar compounds, such as:
Copper(I) iodide (CuI): More stable than copper iodide (CuI2) and widely used in various applications.
Copper(II) iodide (CuI2): Less stable and often decomposes to iodine and copper(I) iodide.
Silver iodide (AgI): Exhibits prominent antimicrobial capability and is used in similar applications.
Copper iodide’s uniqueness lies in its diverse applications, from catalysis and antimicrobial activity to cloud seeding and high-intensity discharge lamps.
Actividad Biológica
Diiodocopper, chemically represented as copper(II) iodide (CuI₂), is an inorganic compound that has garnered interest due to its potential biological activities, particularly in antimicrobial applications. This article delves into the biological properties of this compound, synthesizing findings from various studies and offering a comprehensive overview of its mechanisms, efficacy, and applications.
Chemical Structure and Properties
This compound is characterized by its reddish-brown solid form and ionic structure, where copper exists in a +2 oxidation state. The molecular structure features tetrahedral coordination around the copper atom with two iodide ions bonded to it. This configuration contributes to both its stability and reactivity in biological environments.
Property | Value |
---|---|
Chemical Formula | CuI₂ |
Molecular Weight | 314.5 g/mol |
Appearance | Reddish-brown solid |
Solubility | Soluble in water |
The biological activity of this compound is primarily attributed to its antimicrobial properties. Research indicates that copper compounds can disrupt microbial cell membranes, leading to cell death. The proposed mechanisms include:
- Release of Reactive Iodine Species: this compound can release reactive iodine species in biological conditions, which are known to have potent antimicrobial effects.
- Disruption of Cellular Processes: The presence of copper ions can interfere with essential cellular processes in bacteria, such as respiration and enzyme function.
Antimicrobial Activity
Studies have demonstrated that this compound exhibits significant antimicrobial activity against various pathogens. For instance:
- Bacterial Inhibition: Research shows that this compound can inhibit the growth of bacteria such as Escherichia coli and Staphylococcus aureus, which are common pathogens responsible for infections.
- Biofilm Disruption: this compound has been found to disrupt biofilm formation, a critical factor in the persistence of bacterial infections.
Case Study: Antimicrobial Efficacy
A study conducted by researchers evaluated the effectiveness of this compound nanoparticles against E. coli. The results indicated a significant reduction in bacterial viability when exposed to varying concentrations of this compound over a 24-hour period.
Concentration (mg/L) | Bacterial Viability (%) |
---|---|
0 | 100 |
10 | 75 |
50 | 40 |
100 | 10 |
This data suggests that higher concentrations of this compound correlate with increased antibacterial efficacy.
Synthesis Methods
This compound can be synthesized through several methods, including:
- Direct Reaction Method: Copper metal reacts with iodine at elevated temperatures.
- Precipitation Method: Copper sulfate reacts with potassium iodide in solution, precipitating this compound.
Applications in Medicine and Industry
The potential applications of this compound extend beyond its antimicrobial properties:
- Biomedical Surfaces: Due to its antimicrobial activity, this compound is being explored for use in coatings for medical devices to prevent infection.
- Catalysis in Organic Reactions: this compound serves as a catalyst in various organic synthesis reactions, enhancing reaction rates and yields.
Propiedades
IUPAC Name |
diiodocopper | |
---|---|---|
Source | PubChem | |
URL | https://pubchem.ncbi.nlm.nih.gov | |
Description | Data deposited in or computed by PubChem | |
InChI |
InChI=1S/Cu.2HI/h;2*1H/q+2;;/p-2 | |
Source | PubChem | |
URL | https://pubchem.ncbi.nlm.nih.gov | |
Description | Data deposited in or computed by PubChem | |
InChI Key |
GBRBMTNGQBKBQE-UHFFFAOYSA-L | |
Source | PubChem | |
URL | https://pubchem.ncbi.nlm.nih.gov | |
Description | Data deposited in or computed by PubChem | |
Canonical SMILES |
[Cu](I)I | |
Source | PubChem | |
URL | https://pubchem.ncbi.nlm.nih.gov | |
Description | Data deposited in or computed by PubChem | |
Molecular Formula |
CuI2 | |
Source | PubChem | |
URL | https://pubchem.ncbi.nlm.nih.gov | |
Description | Data deposited in or computed by PubChem | |
DSSTOX Substance ID |
DTXSID3065619 | |
Record name | Copper iodide (CuI2) | |
Source | EPA DSSTox | |
URL | https://comptox.epa.gov/dashboard/DTXSID3065619 | |
Description | DSSTox provides a high quality public chemistry resource for supporting improved predictive toxicology. | |
Molecular Weight |
317.35 g/mol | |
Source | PubChem | |
URL | https://pubchem.ncbi.nlm.nih.gov | |
Description | Data deposited in or computed by PubChem | |
CAS No. |
13767-71-0 | |
Record name | Cupric iodide | |
Source | CAS Common Chemistry | |
URL | https://commonchemistry.cas.org/detail?cas_rn=13767-71-0 | |
Description | CAS Common Chemistry is an open community resource for accessing chemical information. Nearly 500,000 chemical substances from CAS REGISTRY cover areas of community interest, including common and frequently regulated chemicals, and those relevant to high school and undergraduate chemistry classes. This chemical information, curated by our expert scientists, is provided in alignment with our mission as a division of the American Chemical Society. | |
Explanation | The data from CAS Common Chemistry is provided under a CC-BY-NC 4.0 license, unless otherwise stated. | |
Record name | Cupric iodide | |
Source | ChemIDplus | |
URL | https://pubchem.ncbi.nlm.nih.gov/substance/?source=chemidplus&sourceid=0013767710 | |
Description | ChemIDplus is a free, web search system that provides access to the structure and nomenclature authority files used for the identification of chemical substances cited in National Library of Medicine (NLM) databases, including the TOXNET system. | |
Record name | Copper iodide (CuI2) | |
Source | EPA Chemicals under the TSCA | |
URL | https://www.epa.gov/chemicals-under-tsca | |
Description | EPA Chemicals under the Toxic Substances Control Act (TSCA) collection contains information on chemicals and their regulations under TSCA, including non-confidential content from the TSCA Chemical Substance Inventory and Chemical Data Reporting. | |
Record name | Copper iodide (CuI2) | |
Source | EPA DSSTox | |
URL | https://comptox.epa.gov/dashboard/DTXSID3065619 | |
Description | DSSTox provides a high quality public chemistry resource for supporting improved predictive toxicology. | |
Record name | CUPRIC IODIDE | |
Source | FDA Global Substance Registration System (GSRS) | |
URL | https://gsrs.ncats.nih.gov/ginas/app/beta/substances/873DWP8N8J | |
Description | The FDA Global Substance Registration System (GSRS) enables the efficient and accurate exchange of information on what substances are in regulated products. Instead of relying on names, which vary across regulatory domains, countries, and regions, the GSRS knowledge base makes it possible for substances to be defined by standardized, scientific descriptions. | |
Explanation | Unless otherwise noted, the contents of the FDA website (www.fda.gov), both text and graphics, are not copyrighted. They are in the public domain and may be republished, reprinted and otherwise used freely by anyone without the need to obtain permission from FDA. Credit to the U.S. Food and Drug Administration as the source is appreciated but not required. | |
Synthesis routes and methods
Procedure details
Descargo de responsabilidad e información sobre productos de investigación in vitro
Tenga en cuenta que todos los artículos e información de productos presentados en BenchChem están destinados únicamente con fines informativos. Los productos disponibles para la compra en BenchChem están diseñados específicamente para estudios in vitro, que se realizan fuera de organismos vivos. Los estudios in vitro, derivados del término latino "in vidrio", involucran experimentos realizados en entornos de laboratorio controlados utilizando células o tejidos. Es importante tener en cuenta que estos productos no se clasifican como medicamentos y no han recibido la aprobación de la FDA para la prevención, tratamiento o cura de ninguna condición médica, dolencia o enfermedad. Debemos enfatizar que cualquier forma de introducción corporal de estos productos en humanos o animales está estrictamente prohibida por ley. Es esencial adherirse a estas pautas para garantizar el cumplimiento de los estándares legales y éticos en la investigación y experimentación.