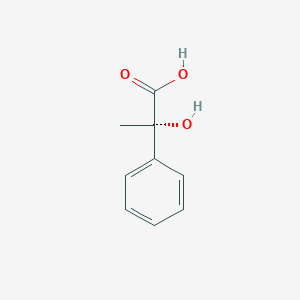
(s)-Atrolactic acid
Descripción general
Descripción
Synthesis Analysis
The synthesis of (S)-Atrolactic acid has been explored through various methods. Notably, a novel approach using Ce–Zr binary oxides as catalysts for the oxidation of α-methylstyrene oxide into atrolactic acid was developed, offering high activity and potential for recycling under benign conditions (Huang et al., 2020). Additionally, asymmetric synthesis evaluations using chiral inducers have demonstrated the capability to achieve high diastereomeric and enantiomeric excesses, showcasing the versatility and efficiency in synthesizing atrolactic acid (Hassine et al., 2010).
Molecular Structure Analysis
The molecular structure of (S)-Atrolactic acid is crucial for its reactivity and application in pharmaceutical synthesis. Its structure allows for the introduction of asymmetry in chemical reactions, facilitating the production of optically active compounds. The efficiency of asymmetric electrochemical carboxylation of prochiral acetophenone to produce (S)-Atrolactic acid underlines the importance of molecular structure in achieving high enantiomeric excesses through selective fixation of carbon dioxide (Zhang et al., 2009).
Aplicaciones Científicas De Investigación
Catalysis Using Cerium–Zirconium Binary Oxide : Huang et al. (2020) explored the use of Ce–Zr binary oxides as catalysts for the oxidation of α-methylstyrene oxide into atrolactic acid, demonstrating high activity and recycling potential. This provides a new, benign method for preparing atrolactic acid with satisfactory outputs (Huang et al., 2020).
Asymmetric Synthesis Using Sugar Derivatives : Kawana and Emoto (1967) investigated the asymmetric synthesis of atrolactic acid using reactions of methylmagnesium iodide with phenylglyoxylic ester and sugar derivatives. The study found that the resulting sugar atrolactates could easily hydrolyze to afford levorotatory acid in 22–38% optical yield (Kawana & Emoto, 1967).
Chiral Induction in Atrolactic Acid Synthesis : Tamai et al. (1992) investigated the chiral induction ability of axially chiral 1,1'-binaphthalene framework in the Prelog's atrolactic acid synthesis. A revised steric model was proposed to explain the selectivity observed in the synthesis (Tamai et al., 1992).
Electrochemical Carboxylation of Acetophenone : Zhang et al. (2009) developed a method for selective fixation of carbon dioxide, producing atrolactic acid from prochiral acetophenone in the presence of chiral alkaloids. This novel approach offers an efficient route to optically active atrolactic acid (Zhang et al., 2009).
Chemoenzymatic Preparation Using Aspergillus Oryzae Protease : Feichter et al. (1991) utilized a protease from Aspergillus oryzae for the chemoenzymatic preparation of atrolactic and Mosher's acid. This method allowed for the convenient resolution of sterically demanding α-methyl- and α-trifluoromethyl-mandelic esters to yield both enantiomers of atrolactic acid with high enantiomeric purity (Feichter et al., 1991).
High-Performance Liquid Chromatographic Enantioseparation : Zhang et al. (2014) established a method for the enantioseparation of atrolactic acids using high-performance liquid chromatography. This method proves to be simple and economical for analytical enantioseparation of atrolactic acid with high peak resolution (Zhang et al., 2014).
Propiedades
IUPAC Name |
(2S)-2-hydroxy-2-phenylpropanoic acid | |
---|---|---|
Source | PubChem | |
URL | https://pubchem.ncbi.nlm.nih.gov | |
Description | Data deposited in or computed by PubChem | |
InChI |
InChI=1S/C9H10O3/c1-9(12,8(10)11)7-5-3-2-4-6-7/h2-6,12H,1H3,(H,10,11)/t9-/m0/s1 | |
Source | PubChem | |
URL | https://pubchem.ncbi.nlm.nih.gov | |
Description | Data deposited in or computed by PubChem | |
InChI Key |
NWCHELUCVWSRRS-VIFPVBQESA-N | |
Source | PubChem | |
URL | https://pubchem.ncbi.nlm.nih.gov | |
Description | Data deposited in or computed by PubChem | |
Canonical SMILES |
CC(C1=CC=CC=C1)(C(=O)O)O | |
Source | PubChem | |
URL | https://pubchem.ncbi.nlm.nih.gov | |
Description | Data deposited in or computed by PubChem | |
Isomeric SMILES |
C[C@](C1=CC=CC=C1)(C(=O)O)O | |
Source | PubChem | |
URL | https://pubchem.ncbi.nlm.nih.gov | |
Description | Data deposited in or computed by PubChem | |
Molecular Formula |
C9H10O3 | |
Source | PubChem | |
URL | https://pubchem.ncbi.nlm.nih.gov | |
Description | Data deposited in or computed by PubChem | |
Molecular Weight |
166.17 g/mol | |
Source | PubChem | |
URL | https://pubchem.ncbi.nlm.nih.gov | |
Description | Data deposited in or computed by PubChem | |
CAS RN |
13113-71-8 | |
Record name | Atrolactic acid, (+)- | |
Source | ChemIDplus | |
URL | https://pubchem.ncbi.nlm.nih.gov/substance/?source=chemidplus&sourceid=0013113718 | |
Description | ChemIDplus is a free, web search system that provides access to the structure and nomenclature authority files used for the identification of chemical substances cited in National Library of Medicine (NLM) databases, including the TOXNET system. | |
Record name | (S)-atrolactic acid | |
Source | DrugBank | |
URL | https://www.drugbank.ca/drugs/DB07381 | |
Description | The DrugBank database is a unique bioinformatics and cheminformatics resource that combines detailed drug (i.e. chemical, pharmacological and pharmaceutical) data with comprehensive drug target (i.e. sequence, structure, and pathway) information. | |
Explanation | Creative Common's Attribution-NonCommercial 4.0 International License (http://creativecommons.org/licenses/by-nc/4.0/legalcode) | |
Record name | ATROLACTIC ACID, (+)- | |
Source | FDA Global Substance Registration System (GSRS) | |
URL | https://gsrs.ncats.nih.gov/ginas/app/beta/substances/22Y6G519RU | |
Description | The FDA Global Substance Registration System (GSRS) enables the efficient and accurate exchange of information on what substances are in regulated products. Instead of relying on names, which vary across regulatory domains, countries, and regions, the GSRS knowledge base makes it possible for substances to be defined by standardized, scientific descriptions. | |
Explanation | Unless otherwise noted, the contents of the FDA website (www.fda.gov), both text and graphics, are not copyrighted. They are in the public domain and may be republished, reprinted and otherwise used freely by anyone without the need to obtain permission from FDA. Credit to the U.S. Food and Drug Administration as the source is appreciated but not required. | |
Synthesis routes and methods I
Procedure details
Synthesis routes and methods II
Procedure details
Synthesis routes and methods III
Procedure details
Retrosynthesis Analysis
AI-Powered Synthesis Planning: Our tool employs the Template_relevance Pistachio, Template_relevance Bkms_metabolic, Template_relevance Pistachio_ringbreaker, Template_relevance Reaxys, Template_relevance Reaxys_biocatalysis model, leveraging a vast database of chemical reactions to predict feasible synthetic routes.
One-Step Synthesis Focus: Specifically designed for one-step synthesis, it provides concise and direct routes for your target compounds, streamlining the synthesis process.
Accurate Predictions: Utilizing the extensive PISTACHIO, BKMS_METABOLIC, PISTACHIO_RINGBREAKER, REAXYS, REAXYS_BIOCATALYSIS database, our tool offers high-accuracy predictions, reflecting the latest in chemical research and data.
Strategy Settings
Precursor scoring | Relevance Heuristic |
---|---|
Min. plausibility | 0.01 |
Model | Template_relevance |
Template Set | Pistachio/Bkms_metabolic/Pistachio_ringbreaker/Reaxys/Reaxys_biocatalysis |
Top-N result to add to graph | 6 |
Feasible Synthetic Routes
Q & A
Q1: What are the current synthetic approaches to obtain enantiomerically pure (S)-atrolactic acid?
A1: While (S)-atrolactic acid has been studied extensively, accessing it in high enantiomeric purity can be challenging. The provided research highlights two different approaches:
- Asymmetric synthesis using chiral auxiliary: One paper [] describes a method utilizing (S)-(+)-3-hydroxytetrahydrofuran as a chiral auxiliary. This approach achieves an enantiomeric excess (ee) of 20% for (S)-atrolactic acid. While this method demonstrates the potential of using chiral auxiliaries, further optimization is needed to enhance the enantioselectivity.
- Resolution of racemic mixtures: Another study, not included in the provided excerpts but mentioned as a prominent example [], explored the resolution of racemic atrolactic acid by Prelog. While the specifics of this method aren't detailed here, it highlights an alternative strategy for obtaining enantiopure (S)-atrolactic acid.
Q2: What are the limitations of the current synthetic methods for (S)-atrolactic acid, and what are potential future directions for improvement?
A2: The provided research [] highlights the limitation of achieving high enantioselectivity in the asymmetric synthesis of (S)-atrolactic acid using (S)-(+)-3-hydroxytetrahydrofuran. The reported ee of 20% indicates significant room for improvement.
Descargo de responsabilidad e información sobre productos de investigación in vitro
Tenga en cuenta que todos los artículos e información de productos presentados en BenchChem están destinados únicamente con fines informativos. Los productos disponibles para la compra en BenchChem están diseñados específicamente para estudios in vitro, que se realizan fuera de organismos vivos. Los estudios in vitro, derivados del término latino "in vidrio", involucran experimentos realizados en entornos de laboratorio controlados utilizando células o tejidos. Es importante tener en cuenta que estos productos no se clasifican como medicamentos y no han recibido la aprobación de la FDA para la prevención, tratamiento o cura de ninguna condición médica, dolencia o enfermedad. Debemos enfatizar que cualquier forma de introducción corporal de estos productos en humanos o animales está estrictamente prohibida por ley. Es esencial adherirse a estas pautas para garantizar el cumplimiento de los estándares legales y éticos en la investigación y experimentación.