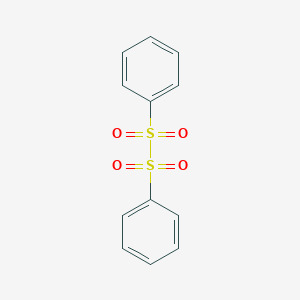
Difenil sulfona
Descripción general
Descripción
Diphenyl disulfone is an organosulfur compound with the chemical formula (C_{12}H_{10}O_{4}S_{2}). It is a white solid that is soluble in organic solvents. This compound is known for its applications in various fields, including chemistry, biology, medicine, and industry. It is often used as a high-temperature solvent and in the synthesis of other chemical compounds.
Aplicaciones Científicas De Investigación
Diphenyl disulfone has a wide range of applications in scientific research:
Chemistry: It is used as a reagent in organic synthesis and as a high-temperature solvent for processing rigid polymers.
Biology: It is used in the study of sulfur-containing compounds and their biological activities.
Industry: It is used in the production of polymers, resins, and other industrial materials.
Mecanismo De Acción
Target of Action
Diphenyl disulfone, also known as Dapsone, primarily targets bacterial synthesis of dihydrofolic acid . It competes with para-aminobenzoate for the active site of dihydropteroate synthase . This enzyme plays a crucial role in the synthesis of folic acid, which is essential for bacterial growth and survival .
Mode of Action
Dapsone inhibits the synthesis of dihydrofolic acid by competing with para-aminobenzoate for the active site of dihydropteroate synthase . This competition inhibits nucleic acid synthesis, thereby preventing bacterial growth . The sulfonamide group of antibacterial drugs also works in this way .
Biochemical Pathways
The primary biochemical pathway affected by Dapsone is the synthesis of dihydrofolic acid . By inhibiting this pathway, Dapsone prevents the formation of folic acid, a vital component for bacterial DNA synthesis and cell division . This inhibition ultimately leads to the death of the bacteria .
Pharmacokinetics
Dapsone has a bioavailability of 70 to 80% and is primarily metabolized in the liver, mostly through CYP2E1-mediated processes . It has a protein binding capacity of 70 to 90% and an elimination half-life of 20 to 30 hours . The primary route of excretion is through the kidneys .
Result of Action
The molecular and cellular effects of Dapsone’s action primarily involve the inhibition of bacterial growth. By preventing the synthesis of dihydrofolic acid, Dapsone disrupts bacterial DNA synthesis and cell division, leading to bacterial death .
Análisis Bioquímico
Biochemical Properties
It is known to be soluble in organic solvents , which suggests it may interact with lipid-based structures within cells
Cellular Effects
Given its solubility in organic solvents , it may influence cell function by interacting with lipid membranes or lipid-based cellular components
Molecular Mechanism
It is produced by the sulfonation of benzene with sulfuric acid and oleum , suggesting it may interact with other molecules through sulfone groups
Métodos De Preparación
Synthetic Routes and Reaction Conditions: Diphenyl disulfone can be synthesized through several methods. One common method involves the oxidation of diphenyl sulfide using oxidizing agents such as hydrogen peroxide or peracids. The reaction typically occurs under mild conditions and yields diphenyl disulfone as the primary product.
Industrial Production Methods: In industrial settings, diphenyl disulfone is produced by the sulfonation of benzene with sulfuric acid and oleum. This process involves the formation of benzenesulfonic acid as an intermediate, which is then further reacted to produce diphenyl disulfone. Another method involves the reaction of benzenesulfonyl chloride with benzene .
Análisis De Reacciones Químicas
Types of Reactions: Diphenyl disulfone undergoes various chemical reactions, including:
Oxidation: It can be oxidized to form sulfone derivatives.
Reduction: It can be reduced to form diphenyl sulfide.
Substitution: It can undergo substitution reactions with nucleophiles, leading to the formation of various substituted derivatives.
Common Reagents and Conditions:
Oxidation: Hydrogen peroxide, peracids.
Reduction: Reducing agents such as lithium aluminum hydride.
Substitution: Nucleophiles such as amines or thiols.
Major Products:
Oxidation: Sulfone derivatives.
Reduction: Diphenyl sulfide.
Substitution: Substituted diphenyl disulfone derivatives.
Comparación Con Compuestos Similares
Diphenyl Sulfone: An organosulfur compound with the formula (C_{12}H_{10}O_{2}S). It is used as a high-temperature solvent and in the synthesis of other chemical compounds.
Dapsone (4,4’-Diaminodiphenyl Sulfone): An antibiotic used in the treatment of leprosy and other bacterial infections.
Comparison:
Diphenyl Disulfone vs. Diphenyl Sulfone: Diphenyl disulfone contains two sulfur atoms, whereas diphenyl sulfone contains only one. This difference in structure leads to variations in their chemical reactivity and applications.
Diphenyl Disulfone vs. Dapsone: While both compounds contain sulfur, dapsone has additional amino groups that confer antibacterial properties.
Actividad Biológica
Diphenyl disulfone (DPS) is a compound that has garnered attention for its various biological activities, particularly in the fields of medicinal chemistry and pharmacology. This article explores the biological activity of DPS, focusing on its antibacterial properties, potential therapeutic applications, and relevant case studies.
Chemical Structure and Properties
Diphenyl disulfone is characterized by its sulfonyl functional groups attached to two phenyl rings. Its chemical formula is , and it exhibits significant stability and solubility in organic solvents. The presence of the sulfonyl group contributes to its reactivity and biological activity.
Antibacterial Activity
DPS has been studied for its antibacterial properties, particularly against various strains of bacteria. Research indicates that diphenyl sulfones, including DPS, demonstrate notable antibacterial effects due to their ability to disrupt bacterial cell walls and inhibit essential metabolic processes.
- Mechanism of Action : The antibacterial activity is believed to stem from the nucleophilicity of the sulfone group, which enhances interaction with bacterial targets. This includes interference with the synthesis of folate, a crucial component for bacterial growth .
- Case Study : A study highlighted that 4,4'-diamino-diphenyl sulfone (DDS), a derivative of DPS, was effective against leprosy-causing bacteria. It was shown to reduce levels of reactive oxygen species, thereby prolonging lifespan and improving mobility in treated subjects .
Anti-Sarcopenic Effects
Recent research has also explored the potential anti-sarcopenic effects of DPS. Sarcopenia refers to the age-related loss of muscle mass and function, which is particularly prevalent among elderly populations.
- Study Findings : A study involving leprosy survivors indicated that those treated with DDS exhibited improved muscle strength and mobility compared to controls. This effect is attributed to the compound's ability to inhibit muscle pyruvate kinase activity and reduce oxidative stress .
Parameter | DDS Group (n=32) | Control Group (n=9) | P-value |
---|---|---|---|
Balance | 2.8 ± 1.2 | 2.0 ± 1.8 | 0.216 |
Walking Speed | 3.3 ± 0.9 | 2.9 ± 0.8 | 0.218 |
Chair Stand | 3.2 ± 1.2 | 2.7 ± 1.3 | 0.308 |
Total Score | 9.3 ± 2.6 | 7.6 ± 3.2 | 0.155 |
Antimalarial Activity
DPS derivatives have been synthesized with enhanced antimalarial properties compared to traditional treatments.
- Research Insights : New analogues derived from DDS have shown superior inhibitory activity against plasmodial enzymes compared to sulfadoxine, a common antimalarial drug . This suggests that DPS could serve as a lead compound for developing new antimalarial therapies.
Environmental Impact and Toxicity
Understanding the environmental impact and potential toxicity of DPS is crucial for its application in pharmaceuticals.
- Toxicological Studies : Investigations into the metabolism and degradation of diphenyl disulfone highlight concerns regarding its accumulation in biological systems and potential environmental hazards .
- Safety Profile : While DPS shows promising biological activities, ongoing studies are necessary to evaluate its long-term safety and environmental effects.
Propiedades
IUPAC Name |
benzenesulfonylsulfonylbenzene | |
---|---|---|
Source | PubChem | |
URL | https://pubchem.ncbi.nlm.nih.gov | |
Description | Data deposited in or computed by PubChem | |
InChI |
InChI=1S/C12H10O4S2/c13-17(14,11-7-3-1-4-8-11)18(15,16)12-9-5-2-6-10-12/h1-10H | |
Source | PubChem | |
URL | https://pubchem.ncbi.nlm.nih.gov | |
Description | Data deposited in or computed by PubChem | |
InChI Key |
CXJVMJWCNFOERL-UHFFFAOYSA-N | |
Source | PubChem | |
URL | https://pubchem.ncbi.nlm.nih.gov | |
Description | Data deposited in or computed by PubChem | |
Canonical SMILES |
C1=CC=C(C=C1)S(=O)(=O)S(=O)(=O)C2=CC=CC=C2 | |
Source | PubChem | |
URL | https://pubchem.ncbi.nlm.nih.gov | |
Description | Data deposited in or computed by PubChem | |
Molecular Formula |
C12H10O4S2 | |
Source | PubChem | |
URL | https://pubchem.ncbi.nlm.nih.gov | |
Description | Data deposited in or computed by PubChem | |
DSSTOX Substance ID |
DTXSID80146273 | |
Record name | Diphenyl disulfone | |
Source | EPA DSSTox | |
URL | https://comptox.epa.gov/dashboard/DTXSID80146273 | |
Description | DSSTox provides a high quality public chemistry resource for supporting improved predictive toxicology. | |
Molecular Weight |
282.3 g/mol | |
Source | PubChem | |
URL | https://pubchem.ncbi.nlm.nih.gov | |
Description | Data deposited in or computed by PubChem | |
CAS No. |
10409-06-0 | |
Record name | Diphenyl disulfone | |
Source | ChemIDplus | |
URL | https://pubchem.ncbi.nlm.nih.gov/substance/?source=chemidplus&sourceid=0010409060 | |
Description | ChemIDplus is a free, web search system that provides access to the structure and nomenclature authority files used for the identification of chemical substances cited in National Library of Medicine (NLM) databases, including the TOXNET system. | |
Record name | Diphenyl disulfone | |
Source | DTP/NCI | |
URL | https://dtp.cancer.gov/dtpstandard/servlet/dwindex?searchtype=NSC&outputformat=html&searchlist=232950 | |
Description | The NCI Development Therapeutics Program (DTP) provides services and resources to the academic and private-sector research communities worldwide to facilitate the discovery and development of new cancer therapeutic agents. | |
Explanation | Unless otherwise indicated, all text within NCI products is free of copyright and may be reused without our permission. Credit the National Cancer Institute as the source. | |
Record name | Diphenyl disulfone | |
Source | EPA DSSTox | |
URL | https://comptox.epa.gov/dashboard/DTXSID80146273 | |
Description | DSSTox provides a high quality public chemistry resource for supporting improved predictive toxicology. | |
Record name | DIPHENYL DISULFONE | |
Source | FDA Global Substance Registration System (GSRS) | |
URL | https://gsrs.ncats.nih.gov/ginas/app/beta/substances/M9F86432DA | |
Description | The FDA Global Substance Registration System (GSRS) enables the efficient and accurate exchange of information on what substances are in regulated products. Instead of relying on names, which vary across regulatory domains, countries, and regions, the GSRS knowledge base makes it possible for substances to be defined by standardized, scientific descriptions. | |
Explanation | Unless otherwise noted, the contents of the FDA website (www.fda.gov), both text and graphics, are not copyrighted. They are in the public domain and may be republished, reprinted and otherwise used freely by anyone without the need to obtain permission from FDA. Credit to the U.S. Food and Drug Administration as the source is appreciated but not required. | |
Retrosynthesis Analysis
AI-Powered Synthesis Planning: Our tool employs the Template_relevance Pistachio, Template_relevance Bkms_metabolic, Template_relevance Pistachio_ringbreaker, Template_relevance Reaxys, Template_relevance Reaxys_biocatalysis model, leveraging a vast database of chemical reactions to predict feasible synthetic routes.
One-Step Synthesis Focus: Specifically designed for one-step synthesis, it provides concise and direct routes for your target compounds, streamlining the synthesis process.
Accurate Predictions: Utilizing the extensive PISTACHIO, BKMS_METABOLIC, PISTACHIO_RINGBREAKER, REAXYS, REAXYS_BIOCATALYSIS database, our tool offers high-accuracy predictions, reflecting the latest in chemical research and data.
Strategy Settings
Precursor scoring | Relevance Heuristic |
---|---|
Min. plausibility | 0.01 |
Model | Template_relevance |
Template Set | Pistachio/Bkms_metabolic/Pistachio_ringbreaker/Reaxys/Reaxys_biocatalysis |
Top-N result to add to graph | 6 |
Feasible Synthetic Routes
Q1: What is the molecular structure of diphenyl disulfone and what are the key bond lengths observed in its crystal structure?
A1: Diphenyl disulfone (C12H10O4S2) features a sulfur-sulfur bond flanked by two sulfonyl groups, each connected to a phenyl ring. X-ray diffraction studies revealed the following average bond lengths: * S-S: 2.193 Å []* S=O: 1.428 Å []* S-C: 1.753 Å []
Q2: How does diphenyl disulfone behave upon exposure to UV light?
A2: Irradiating diphenyl disulfone with UV light triggers its decomposition, yielding a pair of phenylsulfonyl radicals. This photolysis process leads to the formation of various products, including arenesulfonic acids, arenesulfonic anhydrides, thiolsulfonates, diaryl sulfones, biaryls, and sulfur dioxide. []
Descargo de responsabilidad e información sobre productos de investigación in vitro
Tenga en cuenta que todos los artículos e información de productos presentados en BenchChem están destinados únicamente con fines informativos. Los productos disponibles para la compra en BenchChem están diseñados específicamente para estudios in vitro, que se realizan fuera de organismos vivos. Los estudios in vitro, derivados del término latino "in vidrio", involucran experimentos realizados en entornos de laboratorio controlados utilizando células o tejidos. Es importante tener en cuenta que estos productos no se clasifican como medicamentos y no han recibido la aprobación de la FDA para la prevención, tratamiento o cura de ninguna condición médica, dolencia o enfermedad. Debemos enfatizar que cualquier forma de introducción corporal de estos productos en humanos o animales está estrictamente prohibida por ley. Es esencial adherirse a estas pautas para garantizar el cumplimiento de los estándares legales y éticos en la investigación y experimentación.