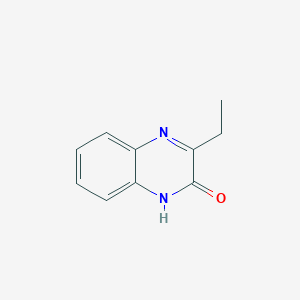
3-Ethylquinoxalin-2(1H)-one
Descripción general
Descripción
3-Ethylquinoxalin-2(1H)-one is a heterocyclic compound that belongs to the quinoxaline family Quinoxalines are known for their diverse biological activities and chemical properties The structure of this compound consists of a quinoxaline core with an ethyl group attached to the third carbon and a keto group at the second position
Análisis Bioquímico
Biochemical Properties
The direct C3-functionalization of 3-Ethylquinoxalin-2(1H)-one via C–H bond activation has been a focus of recent research . This process involves various biochemical reactions, including arylation, alkylation, acylation, alkoxycarbonylation, amination, amidation, and phosphonation
Cellular Effects
The effects of this compound on various types of cells and cellular processes are currently being studied. Preliminary research suggests that it may influence cell function, including impacts on cell signaling pathways, gene expression, and cellular metabolism .
Molecular Mechanism
The molecular mechanism of action of this compound is complex and involves several steps. It is believed to exert its effects at the molecular level through binding interactions with biomolecules, enzyme inhibition or activation, and changes in gene expression .
Temporal Effects in Laboratory Settings
The temporal effects of this compound in laboratory settings are still being explored. Current research is focused on understanding the product’s stability, degradation, and any long-term effects on cellular function observed in in vitro or in vivo studies .
Dosage Effects in Animal Models
Studies on the dosage effects of this compound in animal models are ongoing. These studies aim to understand how the effects of the product vary with different dosages, including any threshold effects observed in these studies, as well as any toxic or adverse effects at high doses .
Metabolic Pathways
This includes any enzymes or cofactors that it interacts with, as well as any effects on metabolic flux or metabolite levels .
Transport and Distribution
Research is ongoing to understand how this compound is transported and distributed within cells and tissues. This includes any transporters or binding proteins that it interacts with, as well as any effects on its localization or accumulation .
Subcellular Localization
The subcellular localization of this compound and any effects on its activity or function are currently being studied. This includes any targeting signals or post-translational modifications that direct it to specific compartments or organelles .
Métodos De Preparación
Synthetic Routes and Reaction Conditions
The synthesis of 3-Ethylquinoxalin-2(1H)-one can be achieved through various methods. One common approach involves the cyclization of o-phenylenediamine with ethyl glyoxalate under acidic conditions. The reaction proceeds through the formation of an intermediate Schiff base, which then undergoes cyclization to form the quinoxaline ring.
Another method involves the direct C3-functionalization of quinoxalin-2(1H)-one via C–H bond activation. This method has gained attention due to its efficiency and the ability to introduce various functional groups at the C3 position .
Industrial Production Methods
Industrial production of this compound typically involves large-scale synthesis using the aforementioned methods. The choice of method depends on factors such as cost, yield, and scalability. The direct C3-functionalization method is particularly attractive for industrial applications due to its simplicity and high yield.
Análisis De Reacciones Químicas
Types of Reactions
3-Ethylquinoxalin-2(1H)-one undergoes various types of chemical reactions, including:
Oxidation: The compound can be oxidized to form quinoxaline-2,3-dione.
Reduction: Reduction of the keto group can yield 3-ethylquinoxalin-2-ol.
Substitution: The ethyl group at the C3 position can be substituted with other functional groups through nucleophilic substitution reactions.
Common Reagents and Conditions
Oxidation: Common oxidizing agents include potassium permanganate and chromium trioxide.
Reduction: Reducing agents such as sodium borohydride and lithium aluminum hydride are commonly used.
Substitution: Nucleophiles such as amines, thiols, and halides can be used for substitution reactions.
Major Products Formed
Oxidation: Quinoxaline-2,3-dione.
Reduction: 3-Ethylquinoxalin-2-ol.
Substitution: Various substituted quinoxalines depending on the nucleophile used.
Aplicaciones Científicas De Investigación
3-Ethylquinoxalin-2(1H)-one has a wide range of applications in scientific research:
Chemistry: It is used as a building block in the synthesis of more complex heterocyclic compounds.
Biology: The compound exhibits antimicrobial and anticancer activities, making it a subject of interest in biological studies.
Medicine: Due to its biological activities, it is being investigated for potential therapeutic applications, including as an anticancer and antimicrobial agent.
Industry: It is used in the development of dyes, pigments, and other industrial chemicals.
Mecanismo De Acción
The mechanism of action of 3-Ethylquinoxalin-2(1H)-one involves its interaction with various molecular targets. In biological systems, it can inhibit the activity of certain enzymes, leading to antimicrobial and anticancer effects. The compound can also interact with DNA, causing disruption of cellular processes and leading to cell death in cancer cells .
Comparación Con Compuestos Similares
Similar Compounds
Quinoxalin-2(1H)-one: The parent compound without the ethyl group.
3-Methylquinoxalin-2(1H)-one: Similar structure with a methyl group instead of an ethyl group.
3-Phenylquinoxalin-2(1H)-one: Similar structure with a phenyl group at the C3 position.
Uniqueness
3-Ethylquinoxalin-2(1H)-one is unique due to the presence of the ethyl group at the C3 position, which can influence its chemical reactivity and biological activity. The ethyl group can enhance the lipophilicity of the compound, potentially improving its ability to penetrate cell membranes and interact with intracellular targets .
Actividad Biológica
3-Ethylquinoxalin-2(1H)-one is a nitrogen-containing heterocyclic compound that has garnered attention in medicinal chemistry due to its diverse biological activities. This article explores the biological activity of this compound, focusing on its anticancer properties, mechanism of action, and potential therapeutic applications.
Synthesis and Structure
The synthesis of this compound typically involves the condensation of ethyl aniline with 1,2-diketones, followed by cyclization. The resulting compound features a quinoxaline core, which is known for its ability to interact with various biological targets.
Anticancer Properties
Numerous studies have documented the anticancer effects of quinoxaline derivatives, including this compound. These compounds exhibit cytotoxicity against various cancer cell lines through different mechanisms:
- Cell Viability Inhibition : In vitro studies have shown that this compound can significantly reduce cell viability in cancer cell lines such as MDA-MB-231 (breast cancer) and PC-3 (prostate cancer). The growth inhibitory concentration (GI50) values for related quinoxaline derivatives range from 28 to 48 µM, indicating a promising potential for further development as anticancer agents .
- Mechanism of Action : The compound appears to induce apoptosis in cancer cells by stabilizing heat shock proteins (Hsp90 and Hsp70) and degrading cyclin-dependent kinase 4 (CDK4), which is crucial for cell cycle progression. This mechanism disrupts the proliferation of cancer cells without triggering a heat shock response, making it a valuable target for therapeutic intervention .
Enzymatic Inhibition
In addition to its anticancer properties, this compound has shown potential as an inhibitor of various enzymes involved in tumor progression:
- Cyclooxygenase-2 (COX-2) : Some quinoxaline derivatives have demonstrated significant inhibition of COX-2, an enzyme associated with inflammation and cancer progression. This inhibition could contribute to the overall anticancer effects observed with these compounds .
- Lactate Dehydrogenase A (LDHA) : LDHA plays a critical role in the metabolic reprogramming of cancer cells. Inhibitors targeting LDHA can effectively reduce lactate production and promote apoptosis in tumor cells .
Case Study 1: In Vitro Evaluation
A study evaluated the antiproliferative activity of several quinoxaline derivatives, including this compound, against MDA-MB-231 and PC-3 cell lines. The results indicated that compounds with specific substituents on the quinoxaline ring showed enhanced cytotoxicity compared to others. The most active derivatives exhibited GI50 values as low as 28 µM against PC-3 cells, highlighting their therapeutic potential .
Compound | Cell Line | GI50 (µM) |
---|---|---|
3b | PC-3 | 28 |
4e | MDA-MB-231 | 32 |
5b | MCF-7 | 45 |
Case Study 2: Mechanistic Insights
Another investigation focused on the mechanistic pathways through which these compounds exert their effects. The study utilized Western blot analysis to assess protein levels of CDK4, Hsp90, and Hsp70 following treatment with quinoxaline derivatives. Results demonstrated a marked decrease in CDK4 levels alongside increased stability of Hsp90 and Hsp70, suggesting a dual mechanism involving both degradation of cell cycle regulators and stabilization of chaperone proteins .
Propiedades
IUPAC Name |
3-ethyl-1H-quinoxalin-2-one | |
---|---|---|
Source | PubChem | |
URL | https://pubchem.ncbi.nlm.nih.gov | |
Description | Data deposited in or computed by PubChem | |
InChI |
InChI=1S/C10H10N2O/c1-2-7-10(13)12-9-6-4-3-5-8(9)11-7/h3-6H,2H2,1H3,(H,12,13) | |
Source | PubChem | |
URL | https://pubchem.ncbi.nlm.nih.gov | |
Description | Data deposited in or computed by PubChem | |
InChI Key |
DZBGIZOIMSCJLV-UHFFFAOYSA-N | |
Source | PubChem | |
URL | https://pubchem.ncbi.nlm.nih.gov | |
Description | Data deposited in or computed by PubChem | |
Canonical SMILES |
CCC1=NC2=CC=CC=C2NC1=O | |
Source | PubChem | |
URL | https://pubchem.ncbi.nlm.nih.gov | |
Description | Data deposited in or computed by PubChem | |
Molecular Formula |
C10H10N2O | |
Source | PubChem | |
URL | https://pubchem.ncbi.nlm.nih.gov | |
Description | Data deposited in or computed by PubChem | |
DSSTOX Substance ID |
DTXSID80344853 | |
Record name | 3-Ethylquinoxalin-2(1H)-one | |
Source | EPA DSSTox | |
URL | https://comptox.epa.gov/dashboard/DTXSID80344853 | |
Description | DSSTox provides a high quality public chemistry resource for supporting improved predictive toxicology. | |
Molecular Weight |
174.20 g/mol | |
Source | PubChem | |
URL | https://pubchem.ncbi.nlm.nih.gov | |
Description | Data deposited in or computed by PubChem | |
Solubility |
>26.1 [ug/mL] (The mean of the results at pH 7.4) | |
Record name | SID26658605 | |
Source | Burnham Center for Chemical Genomics | |
URL | https://pubchem.ncbi.nlm.nih.gov/bioassay/1996#section=Data-Table | |
Description | Aqueous solubility in buffer at pH 7.4 | |
CAS No. |
13297-35-3 | |
Record name | 3-Ethylquinoxalin-2(1H)-one | |
Source | EPA DSSTox | |
URL | https://comptox.epa.gov/dashboard/DTXSID80344853 | |
Description | DSSTox provides a high quality public chemistry resource for supporting improved predictive toxicology. | |
Retrosynthesis Analysis
AI-Powered Synthesis Planning: Our tool employs the Template_relevance Pistachio, Template_relevance Bkms_metabolic, Template_relevance Pistachio_ringbreaker, Template_relevance Reaxys, Template_relevance Reaxys_biocatalysis model, leveraging a vast database of chemical reactions to predict feasible synthetic routes.
One-Step Synthesis Focus: Specifically designed for one-step synthesis, it provides concise and direct routes for your target compounds, streamlining the synthesis process.
Accurate Predictions: Utilizing the extensive PISTACHIO, BKMS_METABOLIC, PISTACHIO_RINGBREAKER, REAXYS, REAXYS_BIOCATALYSIS database, our tool offers high-accuracy predictions, reflecting the latest in chemical research and data.
Strategy Settings
Precursor scoring | Relevance Heuristic |
---|---|
Min. plausibility | 0.01 |
Model | Template_relevance |
Template Set | Pistachio/Bkms_metabolic/Pistachio_ringbreaker/Reaxys/Reaxys_biocatalysis |
Top-N result to add to graph | 6 |
Feasible Synthetic Routes
Q1: What is the significance of 3-Ethylquinoxalin-2(1H)-one in organic synthesis?
A: this compound represents a valuable building block in organic synthesis, particularly for constructing diverse quinoxaline derivatives. Its significance stems from the readily modifiable ethyl group at the 3-position. This allows for the introduction of various functional groups, opening avenues for creating a wide range of structurally diverse compounds. []
Q2: What is a novel and efficient method for synthesizing this compound?
A: A recent study details a new and efficient method for synthesizing this compound. [] The process involves reacting o-phenylenediamine with ethyl 2-oxobutanoate. The ethyl 2-oxobutanoate itself is derived from a Grignard reaction involving diethyl oxalate and either ethylmagnesium bromide or ethylmagnesium iodide. []
Q3: How can the ethyl group in this compound be functionalized?
A: The ethyl group of this compound demonstrates remarkable versatility in its transformation into various functional groups. These include, but are not limited to, α-bromoethyl, α-thiocyanato, α-azidoethyl, α-phenylaminoethyl, acetyl, and bromoacetyl. [] This functionalization significantly expands the structural diversity achievable from this core molecule.
Q4: Can you elaborate on the utility of 3-(bromoacetyl)quinoxalin-2(1H)-one derived from this compound?
A: 3-(bromoacetyl)quinoxalin-2(1H)-one, derived from this compound, serves as a crucial intermediate. Reacting it with either thiourea or hydrazine-1,2-dicarbothioamide leads to the formation of corresponding 3-(2-amino-4-thiazolyl) derivatives. [] These derivatives hold potential for various applications in medicinal chemistry and material science.
Q5: Why is the development of synthetic routes for this compound preferred over 3-(α-chlorobenzyl)quinoxalin-2(1H)-one, especially when the latter is known to be a versatile precursor for diverse fused quinoxaline derivatives?
A: While 3-(α-chlorobenzyl)quinoxalin-2(1H)-one has proven useful in synthesizing fused quinoxaline derivatives, substituting the phenyl group with a methyl group, as seen in this compound, offers significant advantages. [] The α-chloroethyl fragment in this compound is more amenable to transformation into various functional groups, significantly broadening its synthetic utility compared to the 3-(α-chlorobenzyl) analogue. []
Descargo de responsabilidad e información sobre productos de investigación in vitro
Tenga en cuenta que todos los artículos e información de productos presentados en BenchChem están destinados únicamente con fines informativos. Los productos disponibles para la compra en BenchChem están diseñados específicamente para estudios in vitro, que se realizan fuera de organismos vivos. Los estudios in vitro, derivados del término latino "in vidrio", involucran experimentos realizados en entornos de laboratorio controlados utilizando células o tejidos. Es importante tener en cuenta que estos productos no se clasifican como medicamentos y no han recibido la aprobación de la FDA para la prevención, tratamiento o cura de ninguna condición médica, dolencia o enfermedad. Debemos enfatizar que cualquier forma de introducción corporal de estos productos en humanos o animales está estrictamente prohibida por ley. Es esencial adherirse a estas pautas para garantizar el cumplimiento de los estándares legales y éticos en la investigación y experimentación.