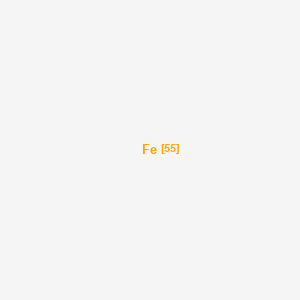
Iron Fe-55
Descripción general
Descripción
Iron-55 is a radioactive isotope of the chemical element iron. It has a nucleus containing 26 protons and 29 neutrons, resulting in a mass number of 55. Iron-55 decays by electron capture to manganese-55, with a half-life of approximately 2.737 years . This isotope is notable for its use in various scientific analysis methods, such as X-ray diffraction and X-ray fluorescence, due to the X-rays emitted during its decay .
Métodos De Preparación
Iron-55 is most effectively produced by neutron irradiation of stable iron isotopes. The reactions involved are:
- (^{54}\text{Fe} + n \rightarrow ^{55}\text{Fe})
- (^{56}\text{Fe} + n \rightarrow ^{55}\text{Fe} + 2n)
These reactions occur when iron-54 and iron-56, the two most abundant isotopes of iron, are exposed to neutron radiation . Industrial production methods typically involve the irradiation of iron-containing components such as steel or reinforced concrete that are exposed to neutron radiation .
Análisis De Reacciones Químicas
Iron-55 undergoes various types of chemical reactions, including:
Oxidation: Iron-55 can be oxidized to form iron oxides.
Reduction: It can be reduced back to its metallic form.
Substitution: Iron-55 can participate in substitution reactions where it replaces another element in a compound.
Common reagents and conditions used in these reactions include hydrochloric acid for reduction and oxygen or air for oxidation . The major products formed from these reactions are iron oxides and metallic iron .
Aplicaciones Científicas De Investigación
Iron-55 has several scientific research applications:
X-ray Diffraction and Fluorescence: The X-rays emitted during the decay of iron-55 are used as a laboratory source for X-ray diffraction and fluorescence techniques.
Electron Capture Detectors: The Auger electrons produced during its decay are used in electron capture detectors for gas chromatography.
Environmental Studies: Iron-55 is used to study the distribution and movement of iron in the environment, particularly in water samples.
Medical Research: It is used in medical research to study iron metabolism and related disorders.
Mecanismo De Acción
Iron-55 decays via electron capture to manganese-55. During this process, an electron from the inner shell of the atom is captured by the nucleus, resulting in the emission of X-rays and Auger electrons . The emitted X-rays are used in various scientific analysis methods, while the Auger electrons are used in electron capture detectors .
Comparación Con Compuestos Similares
Iron-55 can be compared with other radioactive isotopes of iron, such as iron-59. While both isotopes are used in scientific research, iron-55 is primarily used for its X-ray emissions, whereas iron-59 is used for its gamma radiation . Other similar compounds include nickel-63, which is also used in electron capture detectors but emits electrons from beta decay rather than electron capture .
Propiedades
IUPAC Name |
iron-55 | |
---|---|---|
Source | PubChem | |
URL | https://pubchem.ncbi.nlm.nih.gov | |
Description | Data deposited in or computed by PubChem | |
InChI |
InChI=1S/Fe/i1-1 | |
Source | PubChem | |
URL | https://pubchem.ncbi.nlm.nih.gov | |
Description | Data deposited in or computed by PubChem | |
InChI Key |
XEEYBQQBJWHFJM-BJUDXGSMSA-N | |
Source | PubChem | |
URL | https://pubchem.ncbi.nlm.nih.gov | |
Description | Data deposited in or computed by PubChem | |
Canonical SMILES |
[Fe] | |
Source | PubChem | |
URL | https://pubchem.ncbi.nlm.nih.gov | |
Description | Data deposited in or computed by PubChem | |
Isomeric SMILES |
[55Fe] | |
Source | PubChem | |
URL | https://pubchem.ncbi.nlm.nih.gov | |
Description | Data deposited in or computed by PubChem | |
Molecular Formula |
Fe | |
Source | PubChem | |
URL | https://pubchem.ncbi.nlm.nih.gov | |
Description | Data deposited in or computed by PubChem | |
DSSTOX Substance ID |
DTXSID80891771 | |
Record name | Iron 55 | |
Source | EPA DSSTox | |
URL | https://comptox.epa.gov/dashboard/DTXSID80891771 | |
Description | DSSTox provides a high quality public chemistry resource for supporting improved predictive toxicology. | |
Molecular Weight |
54.938291 g/mol | |
Source | PubChem | |
URL | https://pubchem.ncbi.nlm.nih.gov | |
Description | Data deposited in or computed by PubChem | |
CAS No. |
14681-59-5 | |
Record name | Iron Fe-55 | |
Source | ChemIDplus | |
URL | https://pubchem.ncbi.nlm.nih.gov/substance/?source=chemidplus&sourceid=0014681595 | |
Description | ChemIDplus is a free, web search system that provides access to the structure and nomenclature authority files used for the identification of chemical substances cited in National Library of Medicine (NLM) databases, including the TOXNET system. | |
Record name | Iron 55 | |
Source | EPA DSSTox | |
URL | https://comptox.epa.gov/dashboard/DTXSID80891771 | |
Description | DSSTox provides a high quality public chemistry resource for supporting improved predictive toxicology. | |
Record name | IRON FE-55 | |
Source | FDA Global Substance Registration System (GSRS) | |
URL | https://gsrs.ncats.nih.gov/ginas/app/beta/substances/V53IRR2O5Y | |
Description | The FDA Global Substance Registration System (GSRS) enables the efficient and accurate exchange of information on what substances are in regulated products. Instead of relying on names, which vary across regulatory domains, countries, and regions, the GSRS knowledge base makes it possible for substances to be defined by standardized, scientific descriptions. | |
Explanation | Unless otherwise noted, the contents of the FDA website (www.fda.gov), both text and graphics, are not copyrighted. They are in the public domain and may be republished, reprinted and otherwise used freely by anyone without the need to obtain permission from FDA. Credit to the U.S. Food and Drug Administration as the source is appreciated but not required. | |
Descargo de responsabilidad e información sobre productos de investigación in vitro
Tenga en cuenta que todos los artículos e información de productos presentados en BenchChem están destinados únicamente con fines informativos. Los productos disponibles para la compra en BenchChem están diseñados específicamente para estudios in vitro, que se realizan fuera de organismos vivos. Los estudios in vitro, derivados del término latino "in vidrio", involucran experimentos realizados en entornos de laboratorio controlados utilizando células o tejidos. Es importante tener en cuenta que estos productos no se clasifican como medicamentos y no han recibido la aprobación de la FDA para la prevención, tratamiento o cura de ninguna condición médica, dolencia o enfermedad. Debemos enfatizar que cualquier forma de introducción corporal de estos productos en humanos o animales está estrictamente prohibida por ley. Es esencial adherirse a estas pautas para garantizar el cumplimiento de los estándares legales y éticos en la investigación y experimentación.