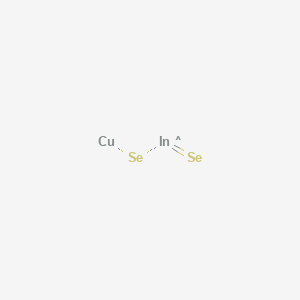
Seleniuro de cobre e indio
- Haga clic en CONSULTA RÁPIDA para recibir una cotización de nuestro equipo de expertos.
- Con productos de calidad a un precio COMPETITIVO, puede centrarse más en su investigación.
Descripción general
Descripción
Copper indium selenide is a semiconducting compound with the chemical formula CuInSe₂. It is a member of the I-III-VI₂ family of semiconductors and is known for its chalcopyrite crystal structure. This compound is primarily used in the production of thin-film solar cells due to its high absorption coefficient and direct bandgap, making it an efficient material for converting sunlight into electricity .
Aplicaciones Científicas De Investigación
Copper indium selenide has a wide range of scientific research applications, including:
Photodetectors: Its high absorption coefficient makes it suitable for use in photodetectors and other optoelectronic devices.
Thermoelectric Devices: Copper indium selenide is also explored for its potential in thermoelectric applications, where it can convert heat into electrical energy.
Quantum Dots: The compound is used to produce quantum dots for applications in bioimaging and solid-state lighting.
Mecanismo De Acción
Target of Action
Copper Indium Selenide (CIS) primarily targets the photovoltaic applications , particularly in the field of solar power generation . It is a key component in thin-film solar cells, which have achieved efficiencies comparable to crystalline silicon (c-Si) wafer-based solar cells .
Mode of Action
CIS interacts with its targets through its unique optical and electronic properties . It is an efficient absorber material for thin-film solar cells . The compound’s interaction with light results in the generation of electric current, a process central to the functioning of solar cells .
Biochemical Pathways
While CIS doesn’t directly participate in biochemical pathways, it plays a crucial role in the energy conversion process in solar cells. When light strikes the CIS layer in a solar cell, it excites electrons, creating electron-hole pairs. These pairs are then separated by an electric field, generating an electric current .
Pharmacokinetics
The term pharmacokinetics typically applies to the absorption, distribution, metabolism, and excretion (ADME) of drugs within a biological systemformation and stability . CIS can be synthesized using various techniques, and its stability is crucial for the long-term performance of solar cells .
Result of Action
The primary result of CIS’s action is the generation of electric current in solar cells. This is achieved through the conversion of solar energy into electrical energy . The efficiency of this energy conversion process is a key measure of the performance of CIS-based solar cells .
Action Environment
The action of CIS is influenced by various environmental factors. For instance, the efficiency of CIS-based solar cells can be affected by the intensity and angle of incident sunlight . Furthermore, the stability and performance of CIS can be influenced by environmental conditions such as temperature and humidity .
Métodos De Preparación
Synthetic Routes and Reaction Conditions: Copper indium selenide can be synthesized through various methods, including co-evaporation, sputtering, and electrodeposition. One common method involves the co-deposition of copper, indium, and selenium onto a substrate, followed by annealing to form the desired compound. The reaction conditions typically involve high temperatures and controlled atmospheres to ensure the correct stoichiometry and phase formation .
Industrial Production Methods: In industrial settings, copper indium selenide is often produced using a two-step process. First, a precursor film of copper and indium is deposited onto a substrate using techniques such as sputtering or evaporation. This is followed by a selenization step, where the precursor film is exposed to selenium vapor at high temperatures to form the final compound .
Análisis De Reacciones Químicas
Types of Reactions: Copper indium selenide undergoes various chemical reactions, including oxidation, reduction, and substitution.
Common Reagents and Conditions:
Oxidation: Copper indium selenide can be oxidized in the presence of oxygen or other oxidizing agents, leading to the formation of copper oxide and indium oxide.
Reduction: Reduction reactions can be carried out using reducing agents such as hydrogen or sodium borohydride, which can convert copper and indium oxides back to their metallic forms.
Major Products Formed: The major products formed from these reactions depend on the specific conditions and reagents used. For example, oxidation typically results in the formation of metal oxides, while reduction can yield pure metals or alloys .
Comparación Con Compuestos Similares
Copper indium selenide is often compared with other similar compounds, such as:
Copper indium gallium selenide: This compound includes gallium in its structure, which allows for tuning of the bandgap and improved efficiency in solar cells.
Copper indium sulfide: By replacing selenium with sulfur, this compound offers different optical and electronic properties, making it suitable for specific applications.
Copper indium telluride: The inclusion of tellurium results in a compound with unique thermoelectric properties.
Copper indium selenide stands out due to its high absorption coefficient, direct bandgap, and versatility in various applications, making it a valuable material in the field of semiconductors and renewable energy .
Propiedades
InChI |
InChI=1S/Cu.In.2Se |
Source
|
---|---|---|
Source | PubChem | |
URL | https://pubchem.ncbi.nlm.nih.gov | |
Description | Data deposited in or computed by PubChem | |
InChI Key |
KTSFMFGEAAANTF-UHFFFAOYSA-N |
Source
|
Source | PubChem | |
URL | https://pubchem.ncbi.nlm.nih.gov | |
Description | Data deposited in or computed by PubChem | |
Canonical SMILES |
[Cu].[Se].[Se]=[In] |
Source
|
Source | PubChem | |
URL | https://pubchem.ncbi.nlm.nih.gov | |
Description | Data deposited in or computed by PubChem | |
Molecular Formula |
CuInSe2 |
Source
|
Source | PubChem | |
URL | https://pubchem.ncbi.nlm.nih.gov | |
Description | Data deposited in or computed by PubChem | |
Molecular Weight |
336.31 g/mol |
Source
|
Source | PubChem | |
URL | https://pubchem.ncbi.nlm.nih.gov | |
Description | Data deposited in or computed by PubChem | |
Descargo de responsabilidad e información sobre productos de investigación in vitro
Tenga en cuenta que todos los artículos e información de productos presentados en BenchChem están destinados únicamente con fines informativos. Los productos disponibles para la compra en BenchChem están diseñados específicamente para estudios in vitro, que se realizan fuera de organismos vivos. Los estudios in vitro, derivados del término latino "in vidrio", involucran experimentos realizados en entornos de laboratorio controlados utilizando células o tejidos. Es importante tener en cuenta que estos productos no se clasifican como medicamentos y no han recibido la aprobación de la FDA para la prevención, tratamiento o cura de ninguna condición médica, dolencia o enfermedad. Debemos enfatizar que cualquier forma de introducción corporal de estos productos en humanos o animales está estrictamente prohibida por ley. Es esencial adherirse a estas pautas para garantizar el cumplimiento de los estándares legales y éticos en la investigación y experimentación.