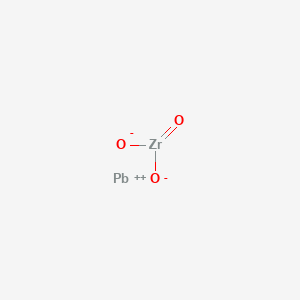
Lead zirconate
Descripción general
Descripción
Lead zirconate (PbZrO₃, PZ) is a perovskite-type ceramic material with antiferroelectric properties at room temperature . It serves as a key component in this compound titanate (PZT), a solid solution of PZ and lead titanate (PbTiO₃, PT). PZ exhibits a pseudocubic orthorhombic structure, distinguishing it from the tetragonal ferroelectric PT and the morphotropic phase boundary (MPB)-driven PZT . Its applications span composites, sensors, and energy-efficient actuators, though its standalone piezoelectric performance is lower than that of PZT .
Métodos De Preparación
Solid-State Synthesis of Lead Zirconate
Process Overview
Solid-state synthesis remains a conventional approach for producing PbZrO₃, involving the high-temperature reaction of lead oxide (PbO) and zirconium dioxide (ZrO₂). The process typically requires mechanical mixing of stoichiometric ratios of raw powders, followed by calcination at temperatures exceeding 1200°C to achieve phase-pure perovskite structures . Excess PbO (5–10 wt.%) is often added to compensate for lead volatility during sintering .
Synthesis Parameters and Challenges
Thermogravimetric analyses reveal that PbO sublimation becomes significant above 800°C, necessitating rapid heating rates and sealed environments to minimize compositional deviations . A study employing shaker milling prior to calcination demonstrated that pre-mixing enhances reactant homogeneity, reducing the required sintering temperature to 900°C while maintaining a bimodal particle size distribution of 5–20 μm . However, prolonged processing at high temperatures often results in coarse, agglomerated particles, limiting the method’s suitability for applications requiring nanoscale morphologies .
Phase Purity and Morphological Characteristics
X-ray diffraction (XRD) patterns of solid-state-derived PbZrO₃ confirmed perovskite crystallization at 1200°C, with secondary PbO phases detected in samples lacking excess lead . Scanning electron microscopy (SEM) images revealed irregularly shaped particles with limited size uniformity, attributed to uncontrolled grain growth during sintering . Despite its simplicity, the method’s high energy consumption and difficulty in achieving sub-micron particle sizes have driven interest in alternative techniques.
Sol-Gel Method for Controlled Stoichiometry
Precursor Selection and Gel Formation
The sol-gel technique offers improved stoichiometric control by utilizing metal alkoxides or nitrates dissolved in organic solvents. For PbZrO₃ synthesis, lead acetate trihydrate (Pb(OAc)₂·3H₂O) and zirconium oxychloride (ZrOCl₂·8H₂O) are commonly dissolved in ethylene glycol, followed by hydrolysis and polycondensation to form a transparent gel . Chelating agents such as citric acid are added to stabilize the solution and prevent premature precipitation .
Thermal Treatment and Crystallization
Dried gels undergo calcination at 700°C for 6 hours, a significant reduction compared to solid-state methods. Fourier-transform infrared (FTIR) spectroscopy studies indicate complete organic residue removal at 500°C, with perovskite phase formation initiating at 600°C . Raman microspectroscopy further confirmed the absence of residual carbonates above 650°C, ensuring high phase purity .
Morphological Advantages and Limitations
Sol-gel-derived PbZrO₃ powders exhibit sub-micron particle sizes (200–500 nm) with reduced agglomeration compared to solid-state counterparts . However, the reliance on expensive alkoxide precursors and stringent pH control during gelation increases production costs, rendering the method less scalable for industrial applications .
Homogeneous Precipitation Using Urea
Mechanism of Urea Decomposition
Homogeneous precipitation employs urea (NH₂CONH₂) as a slow-releasing agent for ammonium (NH₄⁺) and carbonate (CO₃²⁻) ions. When heated to 90°C, urea hydrolyzes to generate a uniform pH increase, facilitating the co-precipitation of lead and zirconium hydroxycarbonates . This method avoids localized supersaturation, a common cause of particle size heterogeneity in conventional precipitation .
Calcination and Phase Development
Precipitated precursors are calcined at 700°C for 6 hours, yielding phase-pure PbZrO₃ with a cubic morphology . XRD analysis showed no secondary phases, while energy-dispersive X-ray spectroscopy (EDS) confirmed a Pb:Zr ratio of 1:1 within 2% error . SEM imaging revealed spherical particles with diameters of 0.5–2 μm, though some agglomeration persisted due to residual hydroxyl groups .
Comparative Advantages
The urea-mediated approach eliminates the need for complex equipment or organic solvents, offering a cost-effective route for large-scale synthesis. Additionally, the method’s low calcination temperature minimizes PbO loss, addressing a critical limitation of solid-state processing .
Emerging Techniques and Hybrid Approaches
Hydrothermal Synthesis Adaptations
While hydrothermal methods are widely used for this compound titanate (PZT), recent adaptations for PbZrO₃ involve reacting zirconyl nitrate (ZrO(NO₃)₂) and lead acetate in alkaline solutions at 200°C . A 3-hour processing time with 2M potassium hydroxide (KOH) as a mineralizer yielded nanoparticles (200–800 nm) with high crystallinity, though phase purity requires further optimization .
Spark Plasma Sintering (SPS)
SPS has been explored to densify PbZrO₃ powders at lower temperatures (1000°C) under uniaxial pressure. This technique reduces processing time to 10–15 minutes while achieving 95% theoretical density, though equipment costs remain prohibitive for widespread adoption .
Critical Comparison of Synthesis Methods
Table 1: Comparative Analysis of PbZrO₃ Preparation Techniques
Análisis De Reacciones Químicas
Thermal Decomposition of Lead Zirconyl Oxalate
A patented method involves synthesizing lead zirconate via thermal decomposition of lead zirconyl oxalate double salts :
-
Precursor Formation : Lead nitrate reacts with zirconyl nitrate in the presence of oxalic acid to form lead zirconyl oxalate:
-
Thermal Decomposition : The oxalate precursor is heated at 500–700°C, yielding this compound:
This method produces high-purity powders (0.5 µm particle size) with a 96% yield .
Parameter | Value |
---|---|
Decomposition Temp. | 700°C (2 hours) |
Particle Size | 0.5 µm |
Yield | 96% |
Crystal Phase | Pure perovskite |
Composition-Dependent Phase Stability
-
Morphotropic Phase Boundary (MPB) : At Zr/Ti = 52/48, PZT exhibits mixed tetragonal and rhombohedral phases, enhancing piezoelectric response .
-
Ti-Rich Compositions : Increased volume fraction of c-domains (tetragonal phase) under biaxial tensile stress enables ferroelastic switching, reducing crack initiation stress to 1137 MPa .
Zr/Ti Ratio | Crystal Phase | Key Property |
---|---|---|
52/48 | Tetragonal + Rhombohedral | High piezoelectric coefficient |
40/60 | Rhombohedral | Enhanced ferroelastic toughening |
30/70 | Tetragonal-dominated | Lower actuation voltage |
Electrode Interface Reactions
This compound reacts with platinum (Pt) electrodes during processing, forming a non-ferroelectric interfacial layer :
This reaction thickens the interfacial layer, degrading polarization switching. In contrast, iridium oxide (IrO₂) electrodes remain inert, preserving ferroelectric properties .
Additive Effects on Reactivity
Aplicaciones Científicas De Investigación
Piezoelectric Devices
Lead zirconate is primarily known for its piezoelectric properties, making it suitable for various sensors and actuators. The following applications highlight its significance:
- Microelectromechanical Systems (MEMS) : this compound titanate (PZT), a solid solution of this compound and lead titanate, is extensively used in MEMS devices. These include accelerometers, energy harvesters, and micropumps, which leverage PZT's high piezoelectric coefficients to convert mechanical stress into electrical energy or vice versa .
- Medical Devices : PZT is utilized in ultrasound transducers and other medical imaging technologies. Its ability to generate and respond to sound waves makes it ideal for non-invasive diagnostic tools .
Energy Harvesting
The potential for this compound in energy harvesting applications is notable. Researchers are exploring ways to convert mechanical energy from human motion into electrical energy using piezoelectric materials like PZT. This could lead to advancements in self-powered devices, such as wearables and smart sensors .
Sensors
This compound's sensitivity to changes in pressure and temperature allows it to be employed in various sensing applications:
- Biosensors : PZT-based sensors can detect biological markers, such as cancer-related proteins, by monitoring changes in resonance frequency or impedance when analytes bind to the sensor surface .
- Environmental Monitoring : These sensors can also be used for detecting pollutants or hazardous materials in the environment, providing real-time data for safety assessments.
Actuators
In actuators, this compound's ability to produce significant displacement under an electric field makes it valuable for precise control applications:
- Micro-actuators : Used in hard disk drives and optical devices, PZT actuators enable rapid movement with high precision .
- Robotics : In robotics, PZT actuators contribute to the development of soft robots that can mimic biological movements due to their flexibility and responsiveness.
Ferroelectric Random Access Memory (FeRAM)
This compound titanate is a critical material in the development of FeRAM technology. FeRAM utilizes the ferroelectric properties of PZT to create non-volatile memory that retains data without power. This technology promises faster access times and lower power consumption compared to traditional memory technologies .
Aerospace and Defense Applications
The military sector has recognized the potential of this compound in advanced systems:
- Guidance Systems : PZT materials are being integrated into munitions and UAVs (Unmanned Aerial Vehicles) for enhanced targeting and navigation capabilities. Their ability to sense environmental changes allows for improved accuracy and reliability in dynamic conditions .
Emerging Research Directions
Recent studies are focusing on enhancing the performance characteristics of this compound through various methods:
- Low-Temperature Processing : Research into low-temperature processing techniques aims to broaden the application range of PZT films, enabling their use in flexible electronics and smart devices without compromising their properties .
- Composite Materials : The development of composite materials incorporating this compound is being explored to enhance mechanical strength while maintaining piezoelectric performance.
Mecanismo De Acción
The mechanism of action of dioxido(oxo)zirconium;lead(2+) involves its ability to interact with various molecular targets. For example, in catalysis, the compound’s structure allows it to facilitate the formation of amide bonds by providing a stable environment for the reaction to occur. The molecular pathways involved include the coordination of substrates to the zirconium centers, which enhances the reaction efficiency .
Comparación Con Compuestos Similares
Comparison with Lead Titanate (PT)
Structural and Phase Differences
- Crystal Structure : PT adopts a tetragonal ferroelectric phase, whereas PZ is orthorhombic and antiferroelectric at room temperature .
- Phase Transition : PT has a Curie temperature (Tc) of ~490°C, while PZ’s Tc is ~230°C, reflecting its lower thermal stability .
Processing Behavior
- Sol-Gel Dynamics : Unhydrolyzed PT sols exhibit larger particle sizes (~11.5 nm) compared to PZ (~1.0 nm). PT hydrolyzes rapidly, forming gels within 24 hours, whereas PZ and PZT show slower hydrolysis rates due to structural stability .
Electromechanical Properties
- Piezoelectricity : PT has moderate piezoelectric coefficients (d₃₃ ~ 190 pC/N), while PZ’s antiferroelectric nature results in negligible piezoelectric activity unless doped or stressed .
Comparison with Lead Zirconate Titanate (PZT)
Morphotropic Phase Boundary (MPB)
PZT’s exceptional piezoelectric properties arise from the MPB near the 52/48 Zr/Ti ratio, where tetragonal and rhombohedral phases coexist. This boundary enhances electromechanical coupling (d₃₃ > 500 pC/N) and permittivity (εᵣ ~ 2000–3000), far surpassing pure PZ or PT .
Processing and Performance
- Particle Size : PZT sols (6.0 nm) bridge the gap between PT and PZ, with hydrolysis behavior closer to PZ .
- Porosity and Piezoelectricity : 1-3 type porous PZT ceramics achieve a 20% higher piezoelectric coefficient (d₃₃) and double the permittivity of 3-3 type structures at equivalent porosity .
Comparison with Other Perovskite Compounds
Barium Titanate (BaTiO₃, BTO)
- Piezoelectricity : BTO has lower d₃₃ (~190 pC/N) and permittivity (εᵣ ~ 1700) compared to PZT but higher than undoped PZ .
- Temperature Stability : BTO’s Tc (~120°C) limits high-temperature applications, whereas PZT operates stably up to ~350°C .
Lanthanum-Doped PZT (PLZT)
PLZT (e.g., Pb₀.₉₂La₀.₀₈(Zr₀.₇Ti₀.₃)₀.₉₈O₃) enhances optical transparency and electro-optic effects, enabling applications in photonic devices—a feature absent in pure PZ .
Composites and Hybrids
- PZ Composites : Acrylic rubber/PZ composites show a two-order conductivity increase at 0.4% PZ loading, suitable for soft actuators .
- PZT/BiT Composites : PZT combined with bismuth titanate (BiT) improves temperature resilience in aerospace sensors, a property unattainable with PZ alone .
Data Tables
Table 1: Structural and Processing Properties
Compound | Crystal Phase (RT) | Particle Size (nm) | Hydrolysis Time (h) | |
---|---|---|---|---|
PbZrO₃ (PZ) | Orthorhombic | 1.0 | >24 | |
PbTiO₃ (PT) | Tetragonal | 11.5 | ~24 | |
PZT (52/48) | MPB (Mixed) | 6.0 | >24 |
Table 2: Electromechanical Performance
Compound | d₃₃ (pC/N) | εᵣ (1 kHz) | Tc (°C) |
---|---|---|---|
PbZrO₃ | ~10 | ~200 | 230 |
PbTiO₃ | ~190 | ~2000 | 490 |
PZT (MPB) | 500–700 | 2000–3000 | 350 |
BTO | ~190 | ~1700 | 120 |
Actividad Biológica
Lead zirconate titanate (PZT), a well-known piezoelectric material, has garnered significant attention due to its unique properties and potential applications in various fields, including biomedical engineering. This article explores the biological activity of PZT, focusing on its interactions with biological systems, potential applications in medicine, and the implications of its use.
Overview of PZT
PZT is a ceramic material with the chemical formula . It exhibits remarkable piezoelectric properties, which enable it to convert mechanical energy into electrical energy and vice versa. These properties make PZT suitable for applications in sensors, actuators, and energy harvesting devices. Recent studies have also investigated its biological interactions, particularly in medical applications.
1. Cell Interactions
PZT has been shown to influence cellular behavior in various studies. For instance, research indicates that PZT-based piezoelectric sensors can measure deformations in neuronal cells, suggesting that they may play a role in neural interfacing technologies . Additionally, PZT's ability to generate electric fields can affect cell adhesion and proliferation, making it a candidate for tissue engineering applications.
2. Microbial Activity
A study investigated the effects of vibrating PZT bimorphs on soil microorganisms. The results demonstrated that these devices could activate microbial populations, enhancing their metabolic activity and biodiversity . Specifically, the research found that the presence of PZT increased the heat release from microbial communities, indicating heightened metabolic processes. The study highlighted how PZT's electric field influences microbial movement and nutrient absorption.
1. Tumor Detection
PZT materials have been explored for their potential in tumor detection through impedance monitoring. By analyzing changes in impedance-frequency responses when subjected to mechanical stress, researchers aim to improve the accuracy of tumor localization and characterization . This application underscores PZT's utility in non-invasive diagnostic techniques.
2. Drug Delivery Systems
The integration of PZT in drug delivery systems has been proposed due to its piezoelectric properties. The material can facilitate controlled drug release by generating localized electric fields that enhance drug diffusion across biological membranes . This method could lead to more effective therapeutic strategies with reduced side effects.
Case Studies
Research Findings
Recent advancements have highlighted both the potential benefits and challenges associated with using PZT in biological contexts:
- Enhanced Sensory Capabilities : PZT-based devices can be used for real-time monitoring of physiological changes, such as blood flow and pressure variations .
- Toxicological Concerns : Despite its benefits, lead content raises concerns regarding toxicity and environmental impact . Research is ongoing to mitigate these risks while harnessing its beneficial properties.
- Microbial Activation : The use of vibrating PZT devices has been linked to increased metabolic rates in soil microorganisms, suggesting potential applications in bioremediation and agricultural enhancement .
Q & A
Basic Research Questions
Q. What are the standard methods for synthesizing lead zirconate ceramics, and how do process parameters influence phase purity?
this compound is typically synthesized via solid-state reaction or sol-gel processing. In sol-gel methods, precursor sols are hydrolyzed under controlled conditions, with parameters such as hydrolysis time, temperature, and precursor ratios directly affecting particle size and phase homogeneity. For example, this compound titanate (PZT) sols exhibit distinct hydrolysis rates compared to pure this compound (PZ) sols, influencing gelation time and final crystallinity . Solid-state synthesis requires high-temperature sintering (e.g., ~1570°C for phase formation), where impurities or deviations in stoichiometry can lead to secondary phases .
Q. How is the crystal structure of this compound determined experimentally?
X-ray diffraction (XRD) and transmission electron microscopy (TEM) are primary techniques for structural characterization. Advanced methods like inelastic X-ray scattering and Brillouin light scattering are used to probe lattice dynamics and phase transitions. For example, incommensurate phase transitions in antiferroelectric this compound were identified using diffuse X-ray scattering, revealing softening of specific lattice modes .
Q. What safety precautions are necessary when handling this compound in laboratory settings?
Due to its high density (7 g/cm³), insolubility in solvents, and potential lead toxicity, researchers must use personal protective equipment (PPE) to avoid inhalation or skin contact. Work should be conducted in fume hoods, and waste disposal must comply with hazardous material protocols .
Q. What spectroscopic techniques are used to characterize this compound's electronic structure?
X-ray photoelectron spectroscopy (XPS) and Raman spectroscopy are employed to analyze bonding and electronic states. Brillouin scattering has also been used to study phonon modes linked to antiferroelectric behavior .
Advanced Research Questions
Q. What causes discrepancies in reported phase transition temperatures of this compound?
Variations in phase transition temperatures (e.g., paraelectric to antiferroelectric) arise from differences in synthesis conditions, impurity levels (e.g., hafnium contamination), and measurement techniques. Flexoelectric coupling and lattice mode softening, as observed in incommensurate phases, further complicate temperature-dependent behavior .
Q. How do researchers analyze nanostructural evolution in this compound sol-gel precursors?
Small-angle X-ray scattering (SAXS) and rheological measurements are critical. For example, Guinier approximation analysis of SAXS data revealed rod-like particles (6–8 nm) in PZ sols, though polydispersity and aggregation can distort results. Core-shell structures speculated in sols were not experimentally confirmed, highlighting limitations in scattering-based models .
Q. How do grain size variations affect dielectric properties in donor-doped this compound ceramics?
Grain size reduction in donor-doped ceramics (e.g., Nb-doped PZT) enhances domain wall mobility, increasing dielectric permittivity. However, excessive grain refinement (<1 µm) can suppress ferroelectricity due to increased internal stress, requiring precise control during sintering .
Q. What methodological challenges arise when modeling antiferroelectric behavior in this compound?
The incommensurate phase in this compound, driven by flexoelectric coupling, defies conventional commensurate lattice models. Computational approaches must account for non-uniform polarization states and mode softening, often requiring density functional theory (DFT) combined with experimental validation via scattering techniques .
Q. How do polydispersity and aggregation in sol-gel precursors impact thin film quality?
Polydisperse sols lead to inhomogeneous particle packing during spin-coating, creating defects in films. For instance, PZ sols with smaller particle sizes (~1 nm) dominate rheological behavior but aggregate unpredictably, necessitating dynamic light scattering (DLS) or TEM for real-time monitoring .
Q. What strategies resolve contradictions between theoretical predictions and experimental observations of this compound's electromechanical response?
Multiscale modeling—combining atomistic simulations (e.g., DFT for lattice dynamics) and continuum models (e.g., phase-field simulations)—bridges gaps between theory and experiments. Experimental validation using high-resolution XRD or piezoresponse force microscopy (PFM) is critical for refining parameters like flexoelectric coefficients .
Propiedades
IUPAC Name |
dioxido(oxo)zirconium;lead(2+) | |
---|---|---|
Source | PubChem | |
URL | https://pubchem.ncbi.nlm.nih.gov | |
Description | Data deposited in or computed by PubChem | |
InChI |
InChI=1S/3O.Pb.Zr/q;2*-1;+2; | |
Source | PubChem | |
URL | https://pubchem.ncbi.nlm.nih.gov | |
Description | Data deposited in or computed by PubChem | |
InChI Key |
RSCSNKLWUCUPDE-UHFFFAOYSA-N | |
Source | PubChem | |
URL | https://pubchem.ncbi.nlm.nih.gov | |
Description | Data deposited in or computed by PubChem | |
Canonical SMILES |
[O-][Zr](=O)[O-].[Pb+2] | |
Source | PubChem | |
URL | https://pubchem.ncbi.nlm.nih.gov | |
Description | Data deposited in or computed by PubChem | |
Molecular Formula |
O3PbZr | |
Source | PubChem | |
URL | https://pubchem.ncbi.nlm.nih.gov | |
Description | Data deposited in or computed by PubChem | |
Molecular Weight |
346 g/mol | |
Source | PubChem | |
URL | https://pubchem.ncbi.nlm.nih.gov | |
Description | Data deposited in or computed by PubChem | |
Descargo de responsabilidad e información sobre productos de investigación in vitro
Tenga en cuenta que todos los artículos e información de productos presentados en BenchChem están destinados únicamente con fines informativos. Los productos disponibles para la compra en BenchChem están diseñados específicamente para estudios in vitro, que se realizan fuera de organismos vivos. Los estudios in vitro, derivados del término latino "in vidrio", involucran experimentos realizados en entornos de laboratorio controlados utilizando células o tejidos. Es importante tener en cuenta que estos productos no se clasifican como medicamentos y no han recibido la aprobación de la FDA para la prevención, tratamiento o cura de ninguna condición médica, dolencia o enfermedad. Debemos enfatizar que cualquier forma de introducción corporal de estos productos en humanos o animales está estrictamente prohibida por ley. Es esencial adherirse a estas pautas para garantizar el cumplimiento de los estándares legales y éticos en la investigación y experimentación.