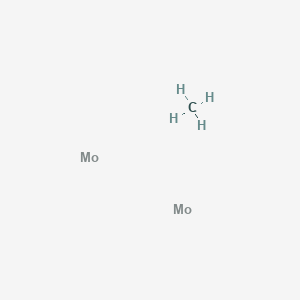
Molybdenum carbide (Mo2C)
Descripción general
Descripción
Molybdenum carbide (Mo₂C) is a transition metal carbide (TMC) belonging to the interstitial carbide family, where carbon atoms occupy octahedral interstitial sites within the molybdenum lattice. It crystallizes in a hexagonal structure (β-Mo₂C) and exhibits exceptional physical and chemical properties, including:
- High melting point (~2690°C) and thermal stability .
- Vickers hardness of ~1950 kg/mm², slightly lower than tungsten carbide (WC) but superior to many metals .
- Catalytic activity comparable to noble metals like platinum, particularly in hydrogen evolution reactions (HER) and hydroprocessing .
- Electronic structure resembling platinum-group metals, enabling efficient charge transfer in electrochemical applications .
Aplicaciones Científicas De Investigación
Catalytic Applications
1.1 Hydrogenation Reactions
Molybdenum carbide exhibits excellent catalytic properties comparable to platinum group metals. It is particularly effective in hydrogenation reactions, where it facilitates the adsorption and dissociation of molecular hydrogen. Studies have shown that Mo₂C can serve as a catalyst for various reactions, including:
- Dehydrogenation of hydrocarbons
- Hydrogenolysis
- Isomerization processes
These reactions benefit from Mo₂C's low cost and high resistance to sulfur poisoning, making it a promising alternative to precious metals in catalytic applications .
1.2 Biomass Conversion
Mo₂C has been explored for its role in converting biomass derivatives into valuable chemicals. Its catalytic properties enable efficient conversion processes, which are crucial for sustainable energy production . For instance, mesoporous carbon-supported Mo₂C has been utilized to enhance the catalytic performance in biomass conversion reactions .
1.3 Water Splitting and Electrocatalysis
Recent advancements have highlighted Mo₂C's potential in electrocatalytic water splitting. Research indicates that doping with non-precious metals such as sulfur or nickel enhances its catalytic activity for hydrogen evolution reactions (HER). This makes Mo₂C a viable candidate for renewable energy applications .
Materials Science Applications
2.1 Coating Materials
Due to its exceptional hardness and wear resistance, Mo₂C is widely used as a coating material in various industrial applications. It is applied through thermal spraying techniques such as:
- Flame spraying
- Plasma spraying
- High-velocity oxygen-fuel (HVOF) spraying
These coatings improve the durability and performance of tools and components exposed to harsh environments .
2.2 Superconductors and Electronic Devices
Two-dimensional (2D) forms of Mo₂C have been investigated for their superconducting properties and potential applications in electronic devices. The unique electronic characteristics of 2D-Mo₂C make it suitable for use in advanced photonic devices and as a mode-locker in ultrafast lasers .
Thermal Stability and Mechanical Properties
Mo₂C exhibits remarkable thermal stability with a melting point of approximately 2692°C, making it suitable for high-temperature applications. Its mechanical properties allow it to withstand extreme conditions without degradation .
Case Studies
Application Area | Description | Key Findings |
---|---|---|
Catalysis | Hydrogenation and biomass conversion | Comparable activity to platinum; effective in biomass conversion processes |
Coatings | Protective coatings for tools and machinery | Enhanced wear resistance; applicable in extreme environments |
Electrocatalysis | Water splitting using Mo₂C-based catalysts | Improved HER activity with non-precious metal doping |
Superconductivity | 2D-Mo₂C in electronic devices | Promising candidate for photonics; tunable properties based on surface treatment |
Mecanismo De Acción
Comparación Con Compuestos Similares
Other Molybdenum Carbide Phases
Mo₂C exists in multiple phases, each with distinct properties:
Key Insight : γ-MoC, though challenging to synthesize in pure form, outperforms β-Mo₂C in acidic HER due to its stable WC-like structure .
Tungsten Carbides (WC, W₂C)
Tungsten carbides are chemically similar but differ in key aspects:
Property | Mo₂C | WC/W₂C |
---|---|---|
Hardness | 1950 kg/mm² | 2080 kg/mm² |
Oxophilicity | Moderate | High |
HER Activity | η₁₀ = 145–198 mV | η₁₀ = 200–250 mV |
Cost | Lower | Higher |
- Synergy in Binary Systems : Mo₂C-W₂C composites enhance catalytic activity in CO₂ reduction and methanation due to W₂C’s oxophilicity improving intermediate adsorption .
Other Transition Metal Carbides (TiC, TaC)
Compound | Melting Point (°C) | Electrical Conductivity | Catalytic Use Case |
---|---|---|---|
Mo₂C | 2690 | High | HER, hydroprocessing |
TiC | 3160 | Moderate | Cutting tools, coatings |
TaC | 3880 | Low | Ultra-high-temp applications |
- Electronic Structure : Mo₂C’s Pt-like density of states near the Fermi level grants superior catalytic versatility compared to TiC/TaC .
MXenes (e.g., Ti₃C₂)
MXenes, 2D carbides/nitrides, share applications with Mo₂C but differ in synthesis and properties:
Property | Mo₂C | Ti₃C₂ (MXene) |
---|---|---|
Synthesis | High-temp reduction | Etching of MAX phase |
Conductivity | Metallic | Metallic |
Flexibility | Brittle | Highly flexible |
HER Activity | η₁₀ = 145 mV | η₁₀ = 200–300 mV |
Mo₂C-based MXenes (e.g., 2D Mo₂C) exhibit enhanced HER performance due to exposed active edges and high surface area .
Molybdenum Nitride (Mo₂N)
Mo₂N, an analog with nitrogen replacing carbon, shows:
Q & A
Basic Research Questions
Q. What are the key thermodynamic considerations in designing solid-state synthesis routes for Mo₂C?
Solid-state synthesis of Mo₂C requires careful control of temperature, carbon source, and oxygen partial pressure. and highlight that Mo₂C formation occurs via direct carburization of MoO₂ at lower temperatures (pseudomorphic transformation) or sequential reduction to metallic Mo followed by carburization at higher temperatures (chemical vapor transport). Phase purity depends on avoiding oxygen contamination, as Mo₂C forms within a narrow compositional range . Thermodynamic calculations (e.g., equilibrium between CO/CO₂ during carburization) are critical to predict reaction pathways and byproducts .
Q. How do reaction mechanisms for Mo₂C synthesis differ between gas-phase and solid-state methods?
Gas-phase methods (e.g., using CH₄ or CO) involve hydrogenation and carburization steps, where MoO₂ reacts with CH₄ or CO to form Mo₂C and H₂O/CO₂ . In contrast, solid-state reactions rely on direct carbon diffusion into Mo lattices. Conflicting mechanisms exist: the Paris Group proposes Mo₂C forms directly from MoO₂ via intermediate oxycarbides (Mo₂CₓOᵧ), while the Stanford Group suggests metallic Mo intermediates . Experimental validation via in situ XRD and mass spectrometry is essential to resolve these pathways .
Q. What characterization techniques are most effective for analyzing Mo₂C phase purity and crystallinity?
X-ray diffraction (XRD) identifies crystalline phases (e.g., β-Mo₂C vs. α-Mo₂C), while Raman spectroscopy detects amorphous carbon residues. Electron microscopy (TEM/SEM) reveals morphology and grain boundaries. Thermogravimetric analysis (TGA) under controlled atmospheres quantifies carburization efficiency . For surface-sensitive studies, X-ray photoelectron spectroscopy (XPS) probes oxidation states of Mo and C .
Advanced Research Questions
Q. How can density functional theory (DFT) guide the design of Mo₂C-based electrocatalysts?
DFT simulations with the Perdew-Burke-Ernzerhof (PBE) functional accurately predict Mo₂C’s electronic structure, surface reactivity, and adsorption energies (e.g., H* for HER). Studies show β-Mo₂C(011) surfaces exhibit metallic character and low work functions (3.4 eV), favoring electron transfer in HER . Hybrid functionals (e.g., HSE06) improve bandgap predictions for semiconductor interfaces like Mo₂C/MoS₂ . Computational workflows should validate against experimental XANES and catalytic performance data .
Q. What in situ techniques resolve the dynamic phase evolution of Mo₂C under catalytic conditions?
In situ XANES/XRD tracks real-time structural changes, such as β-Mo₂C oxidation to Mo₂CₓOᵧ during dry reforming of methane (DRM). and demonstrate that Mo₂C oxidizes to MoO₂ under DRM conditions, correlated with competing reverse water-gas shift (RWGS) activity. Operando diffuse reflectance infrared Fourier transform spectroscopy (DRIFTS) identifies surface intermediates (e.g., adsorbed CO on Mo₂C vs. Ni sites) .
Q. How do heteroatom dopants (e.g., Co, Mn, N, P) optimize Mo₂C’s HER activity?
Doping introduces electronic modulation: Co lowers the d-band center of β-Mo₂C, weakening Mo–H bonding and improving HER kinetics. Mn synergizes with N/P co-dopants to enhance charge transfer and reduce overpotentials (η₁₀ = 125 mV) . Experimental validation involves XPS to confirm dopant incorporation and electrochemical impedance spectroscopy (EIS) to quantify charge-transfer resistance .
Q. What discrepancies exist between computational predictions and experimental observations of Mo₂C stability?
DFT often underestimates Mo₂C’s susceptibility to oxidation under reactive environments. For example, in situ studies reveal rapid β-Mo₂C → Mo₂CₓOᵧ transformation in DRM conditions, despite thermodynamic predictions favoring carbide stability . Multiscale modeling combining ab initio molecular dynamics (AIMD) and microkinetic simulations can bridge this gap .
Q. How do support materials (e.g., carbon, SiO₂) influence Mo₂C’s catalytic performance and stability?
Carbon supports facilitate carburization via CO release, while SiO₂ stabilizes Mo₂C against sintering but promotes oxidation. shows Mo₂C/SiO₂ lacks Mo₂CₓOᵧ intermediates observed in carbon-supported systems, altering DRM pathways. Surface-sensitive techniques like Auger electron spectroscopy (AES) quantify support-carbide interactions .
Q. Methodological Guidance
- For computational studies : Use PAW pseudopotentials and GGA-PBE for bulk properties . Include van der Waals corrections for layered heterostructures (e.g., Mo₂C/MoS₂) .
- For experimental synthesis : Optimize CH₄/H₂ ratios in gas-phase carburization to balance reduction and carbon deposition .
- For catalytic testing : Pair rotating disk electrode (RDE) measurements with in situ Raman to monitor surface reconstruction during HER .
Propiedades
IUPAC Name |
methane;molybdenum | |
---|---|---|
Source | PubChem | |
URL | https://pubchem.ncbi.nlm.nih.gov | |
Description | Data deposited in or computed by PubChem | |
InChI |
InChI=1S/CH4.2Mo/h1H4;; | |
Source | PubChem | |
URL | https://pubchem.ncbi.nlm.nih.gov | |
Description | Data deposited in or computed by PubChem | |
InChI Key |
JAGQSESDQXCFCH-UHFFFAOYSA-N | |
Source | PubChem | |
URL | https://pubchem.ncbi.nlm.nih.gov | |
Description | Data deposited in or computed by PubChem | |
Canonical SMILES |
C.[Mo].[Mo] | |
Source | PubChem | |
URL | https://pubchem.ncbi.nlm.nih.gov | |
Description | Data deposited in or computed by PubChem | |
Molecular Formula |
CH4Mo2 | |
Source | PubChem | |
URL | https://pubchem.ncbi.nlm.nih.gov | |
Description | Data deposited in or computed by PubChem | |
DSSTOX Substance ID |
DTXSID60923529 | |
Record name | Methane--molybdenum (1/2) | |
Source | EPA DSSTox | |
URL | https://comptox.epa.gov/dashboard/DTXSID60923529 | |
Description | DSSTox provides a high quality public chemistry resource for supporting improved predictive toxicology. | |
Molecular Weight |
207.9 g/mol | |
Source | PubChem | |
URL | https://pubchem.ncbi.nlm.nih.gov | |
Description | Data deposited in or computed by PubChem | |
CAS No. |
12069-89-5 | |
Record name | Molybdenum carbide (Mo2C) | |
Source | ChemIDplus | |
URL | https://pubchem.ncbi.nlm.nih.gov/substance/?source=chemidplus&sourceid=0012069895 | |
Description | ChemIDplus is a free, web search system that provides access to the structure and nomenclature authority files used for the identification of chemical substances cited in National Library of Medicine (NLM) databases, including the TOXNET system. | |
Record name | Molybdenum carbide (Mo2C) | |
Source | EPA Chemicals under the TSCA | |
URL | https://www.epa.gov/chemicals-under-tsca | |
Description | EPA Chemicals under the Toxic Substances Control Act (TSCA) collection contains information on chemicals and their regulations under TSCA, including non-confidential content from the TSCA Chemical Substance Inventory and Chemical Data Reporting. | |
Record name | Methane--molybdenum (1/2) | |
Source | EPA DSSTox | |
URL | https://comptox.epa.gov/dashboard/DTXSID60923529 | |
Description | DSSTox provides a high quality public chemistry resource for supporting improved predictive toxicology. | |
Record name | Dimolybdenum carbide | |
Source | European Chemicals Agency (ECHA) | |
URL | https://echa.europa.eu/substance-information/-/substanceinfo/100.031.911 | |
Description | The European Chemicals Agency (ECHA) is an agency of the European Union which is the driving force among regulatory authorities in implementing the EU's groundbreaking chemicals legislation for the benefit of human health and the environment as well as for innovation and competitiveness. | |
Explanation | Use of the information, documents and data from the ECHA website is subject to the terms and conditions of this Legal Notice, and subject to other binding limitations provided for under applicable law, the information, documents and data made available on the ECHA website may be reproduced, distributed and/or used, totally or in part, for non-commercial purposes provided that ECHA is acknowledged as the source: "Source: European Chemicals Agency, http://echa.europa.eu/". Such acknowledgement must be included in each copy of the material. ECHA permits and encourages organisations and individuals to create links to the ECHA website under the following cumulative conditions: Links can only be made to webpages that provide a link to the Legal Notice page. | |
Retrosynthesis Analysis
AI-Powered Synthesis Planning: Our tool employs the Template_relevance Pistachio, Template_relevance Bkms_metabolic, Template_relevance Pistachio_ringbreaker, Template_relevance Reaxys, Template_relevance Reaxys_biocatalysis model, leveraging a vast database of chemical reactions to predict feasible synthetic routes.
One-Step Synthesis Focus: Specifically designed for one-step synthesis, it provides concise and direct routes for your target compounds, streamlining the synthesis process.
Accurate Predictions: Utilizing the extensive PISTACHIO, BKMS_METABOLIC, PISTACHIO_RINGBREAKER, REAXYS, REAXYS_BIOCATALYSIS database, our tool offers high-accuracy predictions, reflecting the latest in chemical research and data.
Strategy Settings
Precursor scoring | Relevance Heuristic |
---|---|
Min. plausibility | 0.01 |
Model | Template_relevance |
Template Set | Pistachio/Bkms_metabolic/Pistachio_ringbreaker/Reaxys/Reaxys_biocatalysis |
Top-N result to add to graph | 6 |
Feasible Synthetic Routes
Descargo de responsabilidad e información sobre productos de investigación in vitro
Tenga en cuenta que todos los artículos e información de productos presentados en BenchChem están destinados únicamente con fines informativos. Los productos disponibles para la compra en BenchChem están diseñados específicamente para estudios in vitro, que se realizan fuera de organismos vivos. Los estudios in vitro, derivados del término latino "in vidrio", involucran experimentos realizados en entornos de laboratorio controlados utilizando células o tejidos. Es importante tener en cuenta que estos productos no se clasifican como medicamentos y no han recibido la aprobación de la FDA para la prevención, tratamiento o cura de ninguna condición médica, dolencia o enfermedad. Debemos enfatizar que cualquier forma de introducción corporal de estos productos en humanos o animales está estrictamente prohibida por ley. Es esencial adherirse a estas pautas para garantizar el cumplimiento de los estándares legales y éticos en la investigación y experimentación.