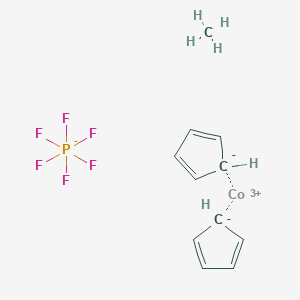
Hexafluorofosfato(1-) de cobaltocenio
- Haga clic en CONSULTA RÁPIDA para recibir una cotización de nuestro equipo de expertos.
- Con productos de calidad a un precio COMPETITIVO, puede centrarse más en su investigación.
Descripción general
Descripción
Cobaltocenium hexafluorophosphate(1-) is an organometallic compound with the formula [Co(C5H5)2]PF6. It is a derivative of cobaltocene, where the cobalt center is in the +3 oxidation state, making it a stable cationic species. This compound is known for its high stability, reversible redox chemistry, and unique electronic properties, making it a valuable material in various scientific and industrial applications.
Aplicaciones Científicas De Investigación
Cobaltocenium hexafluorophosphate(1-) has a wide range of applications in scientific research, including:
Chemistry: It is used as a catalyst in organic reactions, such as the Heck reaction, and as a redox probe in electrochemical studies.
Biology: The compound’s redox properties make it useful in studying biological redox processes and as a potential therapeutic agent.
Medicine: Research is ongoing into its potential use in drug delivery systems and as a component in bioorganometallic compounds.
Industry: Cobaltocenium hexafluorophosphate(1-) is used in the synthesis of functional materials, such as block copolymers and anion exchange membranes.
Mecanismo De Acción
Target of Action
Cobaltocenium hexafluorophosphate, also known as EINECS 235-655-3, is primarily used as a catalyst in various chemical reactions . Its primary targets are the reactants in these reactions, where it facilitates the transformation of these reactants into desired products .
Mode of Action
The compound interacts with its targets through a process known as vicarious nucleophilic substitution . In this process, the cobaltocenium hexafluorophosphate molecule acts as a nucleophile, replacing a hydrogen atom in the target molecule . This interaction results in the formation of a new compound, aminocobaltocenium hexafluorophosphate .
Biochemical Pathways
For instance, it is used in the Heck reaction of aryl iodides with alkenes, where it acts as a co-catalyst . It is also used in the synthesis and solution self-assembly of side-chain cobaltocenium-containing block copolymers .
Result of Action
The primary result of cobaltocenium hexafluorophosphate’s action is the facilitation of chemical reactions, leading to the formation of desired products . For example, in the Heck reaction, it helps in the formation of a carbon-carbon bond between an aryl iodide and an alkene .
Action Environment
The efficacy and stability of cobaltocenium hexafluorophosphate can be influenced by various environmental factors. For instance, the compound is used as a reactant for modifying organometallic electrode surfaces through cathodic reduction, suggesting that it is stable and active under reduction conditions . .
Métodos De Preparación
Synthetic Routes and Reaction Conditions: Cobaltocenium hexafluorophosphate(1-) can be synthesized through several methods. One common approach involves the oxidation of cobaltocene with an oxidizing agent such as ferric chloride (FeCl3) in the presence of hexafluorophosphoric acid (HPF6). The reaction proceeds as follows:
Co(C5H5)2+FeCl3+HPF6→[Co(C5H5)2]PF6+FeCl2+HCl
Industrial Production Methods: Industrial production of cobaltocenium hexafluorophosphate typically involves large-scale oxidation processes using similar reagents and conditions as described above. The process is optimized for high yield and purity, ensuring the compound’s suitability for various applications.
Análisis De Reacciones Químicas
Types of Reactions: Cobaltocenium hexafluorophosphate(1-) undergoes several types of chemical reactions, including:
Nucleophilic Substitution: The compound can participate in nucleophilic substitution reactions, where nucleophiles replace one of the cyclopentadienyl ligands.
Redox Reactions: It exhibits reversible redox behavior, making it useful in electrochemical applications.
Coordination Chemistry: Cobaltocenium hexafluorophosphate(1-) can form coordination complexes with various ligands.
Common Reagents and Conditions:
Nucleophilic Substitution: Reagents such as trimethylhydrazinium iodide can be used under mild conditions to achieve substitution reactions.
Redox Reactions: Electrochemical cells with appropriate electrodes and solvents are used to study the redox behavior.
Coordination Chemistry: Ligands such as phosphines, amines, and other donor molecules are used to form coordination complexes.
Major Products:
Nucleophilic Substitution: Aminocobaltocenium hexafluorophosphate is a common product.
Redox Reactions: The compound can be reduced to cobaltocene or oxidized further depending on the conditions.
Coordination Chemistry: Various coordination complexes with different ligands are formed.
Comparación Con Compuestos Similares
Ferrocene: [Fe(C5H5)2]
Nickelocene: [Ni(C5H5)2]
Ruthenocene: [Ru(C5H5)2]
Propiedades
Número CAS |
12427-42-8 |
---|---|
Fórmula molecular |
C10H10CoF6P |
Peso molecular |
334.08 g/mol |
Nombre IUPAC |
cobalt(3+);cyclopenta-1,3-diene;hexafluorophosphate |
InChI |
InChI=1S/2C5H5.Co.F6P/c2*1-2-4-5-3-1;;1-7(2,3,4,5)6/h2*1-5H;;/q2*-1;+3;-1 |
Clave InChI |
SIZKRAZLFXUUFQ-UHFFFAOYSA-N |
SMILES |
C.[CH-]1C=CC=C1.[CH-]1C=CC=C1.F[P-](F)(F)(F)(F)F.[Co+3] |
SMILES canónico |
[CH-]1C=CC=C1.[CH-]1C=CC=C1.F[P-](F)(F)(F)(F)F.[Co+3] |
Origen del producto |
United States |
Retrosynthesis Analysis
AI-Powered Synthesis Planning: Our tool employs the Template_relevance Pistachio, Template_relevance Bkms_metabolic, Template_relevance Pistachio_ringbreaker, Template_relevance Reaxys, Template_relevance Reaxys_biocatalysis model, leveraging a vast database of chemical reactions to predict feasible synthetic routes.
One-Step Synthesis Focus: Specifically designed for one-step synthesis, it provides concise and direct routes for your target compounds, streamlining the synthesis process.
Accurate Predictions: Utilizing the extensive PISTACHIO, BKMS_METABOLIC, PISTACHIO_RINGBREAKER, REAXYS, REAXYS_BIOCATALYSIS database, our tool offers high-accuracy predictions, reflecting the latest in chemical research and data.
Strategy Settings
Precursor scoring | Relevance Heuristic |
---|---|
Min. plausibility | 0.01 |
Model | Template_relevance |
Template Set | Pistachio/Bkms_metabolic/Pistachio_ringbreaker/Reaxys/Reaxys_biocatalysis |
Top-N result to add to graph | 6 |
Feasible Synthetic Routes
Descargo de responsabilidad e información sobre productos de investigación in vitro
Tenga en cuenta que todos los artículos e información de productos presentados en BenchChem están destinados únicamente con fines informativos. Los productos disponibles para la compra en BenchChem están diseñados específicamente para estudios in vitro, que se realizan fuera de organismos vivos. Los estudios in vitro, derivados del término latino "in vidrio", involucran experimentos realizados en entornos de laboratorio controlados utilizando células o tejidos. Es importante tener en cuenta que estos productos no se clasifican como medicamentos y no han recibido la aprobación de la FDA para la prevención, tratamiento o cura de ninguna condición médica, dolencia o enfermedad. Debemos enfatizar que cualquier forma de introducción corporal de estos productos en humanos o animales está estrictamente prohibida por ley. Es esencial adherirse a estas pautas para garantizar el cumplimiento de los estándares legales y éticos en la investigación y experimentación.