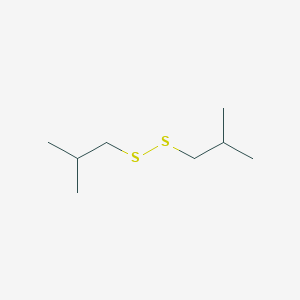
Disulfuro de diisobutilo
Descripción general
Descripción
Diisobutyl disulfide is a useful research compound. Its molecular formula is C8H18S2 and its molecular weight is 178.4 g/mol. The purity is usually 95%.
BenchChem offers high-quality Diisobutyl disulfide suitable for many research applications. Different packaging options are available to accommodate customers' requirements. Please inquire for more information about Diisobutyl disulfide including the price, delivery time, and more detailed information at info@benchchem.com.
Aplicaciones Científicas De Investigación
Material Luminiscente de Detección
El disulfuro de diisobutilo se puede utilizar en el desarrollo de tecnología de detección de material luminiscente . Esta tecnología se ha aplicado ampliamente en la biosensibilidad, el diagnóstico clínico, la seguridad alimentaria y el monitoreo de la contaminación ambiental . Proporciona un análisis cuantitativo, de múltiples componentes con alta sensibilidad y alta especificidad .
Inmunoensayo Enzimático Quimioluminiscente (CL-ELISA)
El this compound se utiliza en el desarrollo de un inmunoensayo enzimático quimioluminiscente (CL-ELISA) para la determinación del ftalato de diisobutilo en agua y alimentos . Este método es conveniente, rápido y económico .
Monitoreo Ambiental
El this compound se puede utilizar en el monitoreo ambiental. Se utiliza en el desarrollo de ensayos para detectar contaminantes en aguas naturales . Las tasas de recuperación del this compound en aguas naturales varían del 79.5 al 113.4 % .
Monitoreo de la Seguridad Alimentaria
El this compound se utiliza en el monitoreo de la seguridad alimentaria. Se utiliza en el desarrollo de ensayos para detectar contaminantes en alimentos, como hojas de lechuga y mariscos . La recuperación del this compound en estas muestras varía en el rango del 76.9 al 134.2 % .
Materiales Blandos Sensibles a Estímulos
El this compound se puede utilizar en el desarrollo de materiales blandos sensibles a estímulos
Mecanismo De Acción
Target of Action
These interactions can alter the function of these proteins and enzymes, leading to various biological effects .
Mode of Action
These free radicals can then react with various cellular components, potentially leading to cellular damage .
Biochemical Pathways
It is known that disulfides can interfere with the function of various enzymes, potentially affecting a wide range of biochemical pathways .
Pharmacokinetics
It is known that disulfides can be absorbed through the skin and mucous membranes, and can be metabolized in the liver .
Result of Action
It is known that disulfides can cause oxidative stress, which can lead to cellular damage .
Action Environment
The action, efficacy, and stability of Diisobutyl disulfide can be influenced by various environmental factors. For example, the presence of reducing agents can influence the reduction of disulfides, potentially affecting their biological activity .
Safety and Hazards
Direcciones Futuras
Disulfide bonds are being targeted for the development of antimicrobial agents because they play a central role in bacterial pathogenesis . The DsbA/DsbB system catalyses disulfide bond formation in a wide array of virulence factors, which are essential for many pathogens to establish infections and cause disease . This makes these redox enzymes well-placed as antimicrobial targets .
Análisis Bioquímico
Biochemical Properties
Disulfide bonds, which Diisobutyl disulfide contains, play a crucial role in the folding and structural stabilization of many important extracellular peptide and protein molecules, including hormones, enzymes, growth factors, toxins, and immunoglobulins . The formation of these bonds requires an oxidizing environment, typically found within the endoplasmic reticulum (ER) of cells .
Cellular Effects
The specific cellular effects of Diisobutyl disulfide are currently unknown. Disulfide bonds, like those found in Diisobutyl disulfide, can have significant impacts on cellular function. They are known to stabilize protein structures and play important roles in protein function and activity . Disruption of these bonds is often associated with a loss of protein function and activity .
Molecular Mechanism
Disulfide bonds are known to play a key role in protein folding, a process that is essential for the proper function of many proteins . The formation of these bonds can help guide the folding process, leading to the correct three-dimensional structure of the protein .
Metabolic Pathways
Disulfide bonds, like those in Diisobutyl disulfide, are known to be involved in various biochemical reactions, particularly those related to protein folding and stabilization .
Subcellular Localization
Proteins with disulfide bonds, like those potentially formed by Diisobutyl disulfide, are typically found within the ER, where the environment is suitable for the formation of these bonds .
Propiedades
IUPAC Name |
2-methyl-1-(2-methylpropyldisulfanyl)propane | |
---|---|---|
Source | PubChem | |
URL | https://pubchem.ncbi.nlm.nih.gov | |
Description | Data deposited in or computed by PubChem | |
InChI |
InChI=1S/C8H18S2/c1-7(2)5-9-10-6-8(3)4/h7-8H,5-6H2,1-4H3 | |
Source | PubChem | |
URL | https://pubchem.ncbi.nlm.nih.gov | |
Description | Data deposited in or computed by PubChem | |
InChI Key |
ONJROLGQWMBXAP-UHFFFAOYSA-N | |
Source | PubChem | |
URL | https://pubchem.ncbi.nlm.nih.gov | |
Description | Data deposited in or computed by PubChem | |
Canonical SMILES |
CC(C)CSSCC(C)C | |
Source | PubChem | |
URL | https://pubchem.ncbi.nlm.nih.gov | |
Description | Data deposited in or computed by PubChem | |
Molecular Formula |
C8H18S2 | |
Source | PubChem | |
URL | https://pubchem.ncbi.nlm.nih.gov | |
Description | Data deposited in or computed by PubChem | |
DSSTOX Substance ID |
DTXSID00164873 | |
Record name | Diisobutyl disulfide | |
Source | EPA DSSTox | |
URL | https://comptox.epa.gov/dashboard/DTXSID00164873 | |
Description | DSSTox provides a high quality public chemistry resource for supporting improved predictive toxicology. | |
Molecular Weight |
178.4 g/mol | |
Source | PubChem | |
URL | https://pubchem.ncbi.nlm.nih.gov | |
Description | Data deposited in or computed by PubChem | |
CAS No. |
1518-72-5 | |
Record name | Bis(2-methylpropyl) disulfide | |
Source | CAS Common Chemistry | |
URL | https://commonchemistry.cas.org/detail?cas_rn=1518-72-5 | |
Description | CAS Common Chemistry is an open community resource for accessing chemical information. Nearly 500,000 chemical substances from CAS REGISTRY cover areas of community interest, including common and frequently regulated chemicals, and those relevant to high school and undergraduate chemistry classes. This chemical information, curated by our expert scientists, is provided in alignment with our mission as a division of the American Chemical Society. | |
Explanation | The data from CAS Common Chemistry is provided under a CC-BY-NC 4.0 license, unless otherwise stated. | |
Record name | Diisobutyl disulfide | |
Source | ChemIDplus | |
URL | https://pubchem.ncbi.nlm.nih.gov/substance/?source=chemidplus&sourceid=0001518725 | |
Description | ChemIDplus is a free, web search system that provides access to the structure and nomenclature authority files used for the identification of chemical substances cited in National Library of Medicine (NLM) databases, including the TOXNET system. | |
Record name | Diisobutyl disulfide | |
Source | EPA DSSTox | |
URL | https://comptox.epa.gov/dashboard/DTXSID00164873 | |
Description | DSSTox provides a high quality public chemistry resource for supporting improved predictive toxicology. | |
Record name | Diisobutyl disulphide | |
Source | European Chemicals Agency (ECHA) | |
URL | https://echa.europa.eu/substance-information/-/substanceinfo/100.014.707 | |
Description | The European Chemicals Agency (ECHA) is an agency of the European Union which is the driving force among regulatory authorities in implementing the EU's groundbreaking chemicals legislation for the benefit of human health and the environment as well as for innovation and competitiveness. | |
Explanation | Use of the information, documents and data from the ECHA website is subject to the terms and conditions of this Legal Notice, and subject to other binding limitations provided for under applicable law, the information, documents and data made available on the ECHA website may be reproduced, distributed and/or used, totally or in part, for non-commercial purposes provided that ECHA is acknowledged as the source: "Source: European Chemicals Agency, http://echa.europa.eu/". Such acknowledgement must be included in each copy of the material. ECHA permits and encourages organisations and individuals to create links to the ECHA website under the following cumulative conditions: Links can only be made to webpages that provide a link to the Legal Notice page. | |
Record name | DIISOBUTYL DISULFIDE | |
Source | FDA Global Substance Registration System (GSRS) | |
URL | https://gsrs.ncats.nih.gov/ginas/app/beta/substances/Z1G437CRNK | |
Description | The FDA Global Substance Registration System (GSRS) enables the efficient and accurate exchange of information on what substances are in regulated products. Instead of relying on names, which vary across regulatory domains, countries, and regions, the GSRS knowledge base makes it possible for substances to be defined by standardized, scientific descriptions. | |
Explanation | Unless otherwise noted, the contents of the FDA website (www.fda.gov), both text and graphics, are not copyrighted. They are in the public domain and may be republished, reprinted and otherwise used freely by anyone without the need to obtain permission from FDA. Credit to the U.S. Food and Drug Administration as the source is appreciated but not required. | |
Retrosynthesis Analysis
AI-Powered Synthesis Planning: Our tool employs the Template_relevance Pistachio, Template_relevance Bkms_metabolic, Template_relevance Pistachio_ringbreaker, Template_relevance Reaxys, Template_relevance Reaxys_biocatalysis model, leveraging a vast database of chemical reactions to predict feasible synthetic routes.
One-Step Synthesis Focus: Specifically designed for one-step synthesis, it provides concise and direct routes for your target compounds, streamlining the synthesis process.
Accurate Predictions: Utilizing the extensive PISTACHIO, BKMS_METABOLIC, PISTACHIO_RINGBREAKER, REAXYS, REAXYS_BIOCATALYSIS database, our tool offers high-accuracy predictions, reflecting the latest in chemical research and data.
Strategy Settings
Precursor scoring | Relevance Heuristic |
---|---|
Min. plausibility | 0.01 |
Model | Template_relevance |
Template Set | Pistachio/Bkms_metabolic/Pistachio_ringbreaker/Reaxys/Reaxys_biocatalysis |
Top-N result to add to graph | 6 |
Feasible Synthetic Routes
Q1: What is the molecular formula, weight, and relevant spectroscopic data for diisobutyl disulfide?
A1: Diisobutyl disulfide has the molecular formula C8H18S2 and a molecular weight of 178.36 g/mol. While the provided abstracts don't detail specific spectroscopic data for diisobutyl disulfide, they highlight the use of vibrational spectroscopy, particularly focusing on the SS and CS stretch frequencies, to analyze its conformational properties. The research uses these frequencies to correlate conformation with spectroscopic data, particularly for diisobutyl disulfide []. This suggests that these vibrational frequencies are key spectroscopic features for this molecule.
Q2: How is computational chemistry being utilized in the study of diisobutyl disulfide?
A2: The research uses ab initio calculations to develop a simplified force field for diethyl disulfide [, ]. This force field can be further applied to study similar molecules like diisobutyl disulfide. By utilizing computational methods, researchers can predict vibrational frequencies and correlate them to different conformations of the disulfide bond. This information is crucial for understanding the molecule's behavior and potential applications.
Q3: Can you elaborate on the significance of studying SS and CS stretch frequencies in disulfides like diisobutyl disulfide?
A3: The SS and CS stretch frequencies are sensitive to the conformation of the disulfide bond, meaning they change depending on the spatial arrangement of atoms around the S-S bond []. By analyzing these frequencies, researchers can gain insights into the molecule's preferred shape and how it might interact with other molecules. This is particularly relevant for understanding the biological activity of disulfide bonds in proteins, as their conformation can significantly influence protein structure and function.
Descargo de responsabilidad e información sobre productos de investigación in vitro
Tenga en cuenta que todos los artículos e información de productos presentados en BenchChem están destinados únicamente con fines informativos. Los productos disponibles para la compra en BenchChem están diseñados específicamente para estudios in vitro, que se realizan fuera de organismos vivos. Los estudios in vitro, derivados del término latino "in vidrio", involucran experimentos realizados en entornos de laboratorio controlados utilizando células o tejidos. Es importante tener en cuenta que estos productos no se clasifican como medicamentos y no han recibido la aprobación de la FDA para la prevención, tratamiento o cura de ninguna condición médica, dolencia o enfermedad. Debemos enfatizar que cualquier forma de introducción corporal de estos productos en humanos o animales está estrictamente prohibida por ley. Es esencial adherirse a estas pautas para garantizar el cumplimiento de los estándares legales y éticos en la investigación y experimentación.