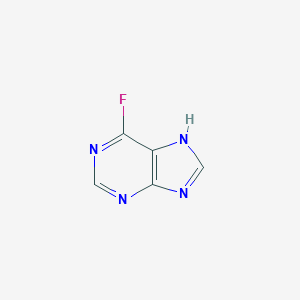
6-Fluoropurine
Descripción general
Descripción
Synthesis Analysis
The synthesis of 6-Fluoropurine has been achieved through various methods. One approach involves the nucleophilic displacement of the trimethylammonio-group of purine with 18F-fluoride under mild conditions . Another method involves the Balz-Schiemann reaction of the corresponding 2-aminopurine starting materials by diazotization-fluorodediazoniation in aqueous fluoroboric acid .Molecular Structure Analysis
The molecular structure of 6-Fluoropurine consists of a purine moiety, which is a pyrimidine-ring fused to an imidazole ring . The compound has a molecular weight of 138.10 g/mol .Chemical Reactions Analysis
The reactions of 6-Fluoropurine are diverse and can be used as synthetic intermediates for medicinal chemistry and reagents for chemical biology studies . The presence of a single fluoro group at the 6-position of a purine can confer advantageous changes to physicochemical, metabolic, and biological properties .Physical And Chemical Properties Analysis
6-Fluoropurine has a molecular weight of 138.10 g/mol, a hydrogen bond donor count of 1, and a hydrogen bond acceptor count of 4 . It has a rotatable bond count of 0, a topological polar surface area of 54.5 Ų, and a heavy atom count of 10 .Aplicaciones Científicas De Investigación
Biomedical Research
Fluorinated purines, including 6-Fluoropurine, have been used extensively in biomedical research . The presence of a single fluoro group at the 6-position of a purine can confer advantageous changes to physicochemical, metabolic, and biological properties .
Medicinal Chemistry
The molecules of fluorinated purines are useful as synthetic intermediates for medicinal chemistry . They are involved in a range of nucleophilic displacements, which make them valuable reagents for chemical biology studies .
Positron Emission Tomography (PET)
The incorporation of an 18 F label into purine nucleosides, including 6-Fluoropurine, provides tools for in vivo imaging by positron emission tomography (PET) . This allows for the visualization of physiological processes in the body.
Anticancer Research
Purine and pyrimidine heterocyclic compounds, including 6-Fluoropurine, have received attention due to their potential in targeting various cancers . The incorporation of the purine ring in synthesized derivatives has resulted in the development of potent anticancer molecules .
Drug Design and Discovery
Several new alternative therapeutic strategies have been tried for the drug design and discovery using purine and pyrimidine heterocyclic compounds, including 6-Fluoropurine . These compounds have shown significantly enhanced anticancer activities against targeted receptor proteins .
Mitigating Drug Resistance
Purine and pyrimidines combined with other heterocyclic compounds have resulted in many novel anticancer molecules that address the challenges of drug resistance . This includes 6-Fluoropurine, which has shown potential in this area .
Safety and Hazards
Direcciones Futuras
Fluorinated purines, including 6-Fluoropurine, have evolved substantially in their chemistry over the past 60 years . They have major applications in biomedical research, and their uses as synthetic intermediates are being explored . The development of structure–function relationships between material properties and biological performance is a promising area of future research .
Mecanismo De Acción
Target of Action
6-Fluoro-7H-purine, also known as 6-Fluoropurine, is a fluorinated purine that exhibits a diverse range of biological activities Fluorinated purines are known to interact with various enzymes and receptors involved in purine metabolism and signaling .
Mode of Action
The mode of action of 6-Fluoropurine involves its interaction with these targets, leading to changes in their physicochemical, metabolic, and biological properties . The presence of a fluoro group at the 6-position of the purine ring can confer advantageous changes to these properties .
Biochemical Pathways
6-Fluoropurine likely affects the purine salvage pathway, a crucial biochemical pathway involved in the recycling of purines
Pharmacokinetics
The presence of a fluoro group can generally enhance the metabolic stability of purine analogs , potentially impacting their bioavailability.
Result of Action
Fluorinated purines are known to exhibit a diverse range of biological activities .
Propiedades
IUPAC Name |
6-fluoro-7H-purine | |
---|---|---|
Source | PubChem | |
URL | https://pubchem.ncbi.nlm.nih.gov | |
Description | Data deposited in or computed by PubChem | |
InChI |
InChI=1S/C5H3FN4/c6-4-3-5(9-1-7-3)10-2-8-4/h1-2H,(H,7,8,9,10) | |
Source | PubChem | |
URL | https://pubchem.ncbi.nlm.nih.gov | |
Description | Data deposited in or computed by PubChem | |
InChI Key |
LGQVOKWMIRXXDM-UHFFFAOYSA-N | |
Source | PubChem | |
URL | https://pubchem.ncbi.nlm.nih.gov | |
Description | Data deposited in or computed by PubChem | |
Canonical SMILES |
C1=NC2=C(N1)C(=NC=N2)F | |
Source | PubChem | |
URL | https://pubchem.ncbi.nlm.nih.gov | |
Description | Data deposited in or computed by PubChem | |
Molecular Formula |
C5H3FN4 | |
Source | PubChem | |
URL | https://pubchem.ncbi.nlm.nih.gov | |
Description | Data deposited in or computed by PubChem | |
DSSTOX Substance ID |
DTXSID40163865 | |
Record name | 6-Fluoropurine | |
Source | EPA DSSTox | |
URL | https://comptox.epa.gov/dashboard/DTXSID40163865 | |
Description | DSSTox provides a high quality public chemistry resource for supporting improved predictive toxicology. | |
Molecular Weight |
138.10 g/mol | |
Source | PubChem | |
URL | https://pubchem.ncbi.nlm.nih.gov | |
Description | Data deposited in or computed by PubChem | |
Product Name |
6-Fluoropurine | |
CAS RN |
1480-89-3 | |
Record name | 6-Fluoropurine | |
Source | ChemIDplus | |
URL | https://pubchem.ncbi.nlm.nih.gov/substance/?source=chemidplus&sourceid=0001480893 | |
Description | ChemIDplus is a free, web search system that provides access to the structure and nomenclature authority files used for the identification of chemical substances cited in National Library of Medicine (NLM) databases, including the TOXNET system. | |
Record name | 6-Fluoropurine | |
Source | EPA DSSTox | |
URL | https://comptox.epa.gov/dashboard/DTXSID40163865 | |
Description | DSSTox provides a high quality public chemistry resource for supporting improved predictive toxicology. | |
Retrosynthesis Analysis
AI-Powered Synthesis Planning: Our tool employs the Template_relevance Pistachio, Template_relevance Bkms_metabolic, Template_relevance Pistachio_ringbreaker, Template_relevance Reaxys, Template_relevance Reaxys_biocatalysis model, leveraging a vast database of chemical reactions to predict feasible synthetic routes.
One-Step Synthesis Focus: Specifically designed for one-step synthesis, it provides concise and direct routes for your target compounds, streamlining the synthesis process.
Accurate Predictions: Utilizing the extensive PISTACHIO, BKMS_METABOLIC, PISTACHIO_RINGBREAKER, REAXYS, REAXYS_BIOCATALYSIS database, our tool offers high-accuracy predictions, reflecting the latest in chemical research and data.
Strategy Settings
Precursor scoring | Relevance Heuristic |
---|---|
Min. plausibility | 0.01 |
Model | Template_relevance |
Template Set | Pistachio/Bkms_metabolic/Pistachio_ringbreaker/Reaxys/Reaxys_biocatalysis |
Top-N result to add to graph | 6 |
Feasible Synthetic Routes
Descargo de responsabilidad e información sobre productos de investigación in vitro
Tenga en cuenta que todos los artículos e información de productos presentados en BenchChem están destinados únicamente con fines informativos. Los productos disponibles para la compra en BenchChem están diseñados específicamente para estudios in vitro, que se realizan fuera de organismos vivos. Los estudios in vitro, derivados del término latino "in vidrio", involucran experimentos realizados en entornos de laboratorio controlados utilizando células o tejidos. Es importante tener en cuenta que estos productos no se clasifican como medicamentos y no han recibido la aprobación de la FDA para la prevención, tratamiento o cura de ninguna condición médica, dolencia o enfermedad. Debemos enfatizar que cualquier forma de introducción corporal de estos productos en humanos o animales está estrictamente prohibida por ley. Es esencial adherirse a estas pautas para garantizar el cumplimiento de los estándares legales y éticos en la investigación y experimentación.