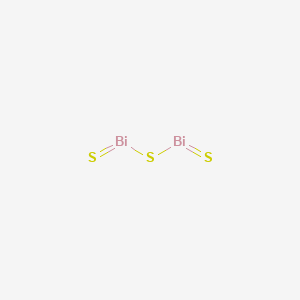
Sulfuro de bismuto (Bi2S3)
Descripción general
Descripción
Bismuth sulfide (Bi2S3) is a chemical compound composed of bismuth and sulfur. It naturally occurs as the mineral bismuthinite, which has a lead-gray streaked color with a metallic luster . Bismuth sulfide is known for its unique properties, including its semiconductor behavior, making it a valuable material in various scientific and industrial applications .
Synthetic Routes and Reaction Conditions:
- Bismuth sulfide can be synthesized by reacting a bismuth (III) salt with hydrogen sulfide. The reaction is as follows:
Reaction with Hydrogen Sulfide: 2Bi3++3H2S→Bi2S3+6H+
Another method involves the reaction of elemental bismuth with elemental sulfur in an evacuated silica tube at 500°C for 96 hours:Reaction of Elemental Bismuth and Sulfur: 2Bi+3S→Bi2S3
Aplicaciones Científicas De Investigación
Optoelectrónica
El sulfuro de bismuto es un semiconductor con una banda prohibida racional alrededor del rango cercano al infrarrojo y visible. Sus nanoestructuras, también conocidas como nano-Bi2S3, han atraído gran atención debido a sus prometedoras actuaciones en materiales y dispositivos optoelectrónicos . Tienen aplicaciones potenciales en fotodetectores, células solares y fotocatalizadores .
Fotodetectores de banda ancha
Las nanocintas de Bi2S3 exhiben un coeficiente de absorción ultraalto en el rango ultravioleta (UV) a infrarrojo cercano (NIR). Los fotodetectores basados en una sola nanocinta de Bi2S3 mostraron un excelente rendimiento de fotorespuesta de banda ancha en el rango UV a NIR (de 300 a 1000 nm), incluida una alta fotoresponsividad de hasta 201 A W −1, una velocidad de respuesta ultarápida de ∼50 μs, una alta eficiencia cuántica externa del 31\u2006140% y una alta detectividad de 2,7 × 10 10 Jones .
Terapia fototérmica
Debido a su superior eficiencia de conversión fototérmica, los nanomateriales basados en Bi2S3 se han utilizado ampliamente en la terapia fototérmica .
Terapia fotodinámica
Los nanomateriales basados en Bi2S3 también se utilizan en la terapia fotodinámica .
Terapia de radiosensibilización
Los nanomateriales basados en Bi2S3 tienen aplicaciones en la terapia de radiosensibilización .
Inmunoterapia
Los nanomateriales basados en Bi2S3 se utilizan en la inmunoterapia .
Quimioterapia
Los nanomateriales basados en Bi2S3 se utilizan en la quimioterapia .
Aplicaciones biomédicas
La investigación biomédica sobre BiNPs ha estado creciendo extensamente y se han explotado varias nanoformas de Bi con diferentes composiciones para aplicaciones biológicas, incluida la administración de fármacos, la actividad antimicrobiana, la bioimagen, la terapia del cáncer, la biosensibilización y la ingeniería de tejidos .
Mecanismo De Acción
Target of Action
Bismuth sulfide (Bi2S3) is a chemical compound of bismuth and sulfur . It primarily targets the gastrointestinal tract when used as a drug . In the field of optoelectronics, it targets light-sensitive applications due to its unique physicochemical properties .
Mode of Action
Bi2S3 interacts with its targets through various mechanisms. In the body, it reacts with naturally occurring sulfides to produce Bismuth (III) sulfide . In optoelectronics, Bi2S3 exhibits a direct band gap semiconductor property, making it useful in light-absorbing applications .
Biochemical Pathways
It’s known that bi2s3 can react with water to supply o2 for the electron on the ti3c2 nss to generate ·o2− and ·oh through electron transfer .
Pharmacokinetics
It’s known that the surface of bi2s3 nanoparticles can be easily modified with biocompatible polymers and proteins, resulting in enhanced colloidal stability, extended blood circulation, and reduced toxicity .
Result of Action
The action of Bi2S3 results in various effects depending on its application. In the body, it can cause temporary black tongue when the sulfides are in the mouth and black feces when the sulfides are in the colon . In optoelectronics, Bi2S3 shows excellent light-to-heat conversion efficiency and a long circulation half-life .
Action Environment
The action of Bi2S3 can be influenced by environmental factors such as temperature and hydrostatic pressure . For instance, the optoelectronic properties of Bi2S3 can be determined under different temperature and hydrostatic pressure conditions .
Análisis Bioquímico
Biochemical Properties
Bismuth Sulfide (Bi2S3) plays a crucial role in biochemical reactions, particularly in the field of photocatalysis . It is widely employed due to its non-toxicity and chemical stability . The interaction of Bismuth Sulfide (Bi2S3) with enzymes, proteins, and other biomolecules is primarily through its photocatalytic activity .
Cellular Effects
The effects of Bismuth Sulfide (Bi2S3) on cells and cellular processes are largely unexplored. Its photocatalytic activity suggests potential influences on cell function, possibly impacting cell signaling pathways, gene expression, and cellular metabolism .
Molecular Mechanism
Its photocatalytic activity suggests that it may exert its effects through binding interactions with biomolecules, potentially influencing enzyme activity and changes in gene expression .
Temporal Effects in Laboratory Settings
Its use in photocatalysis suggests potential long-term effects on cellular function .
Actividad Biológica
Bismuth sulfide (Bi2S3) is a compound that has garnered significant attention in various fields, particularly in biomedicine, due to its unique properties and biological activities. This article delves into the biological activity of Bi2S3, highlighting its synthesis, structural characteristics, and potential applications in therapeutic contexts.
Synthesis and Structural Characteristics
Bismuth sulfide can be synthesized through various methods, including co-precipitation and hydrothermal techniques. The synthesis conditions significantly influence the morphology and size of the nanoparticles, which in turn affect their biological activity.
-
Synthesis Methods :
- Co-precipitation : This method allows for the formation of Bi2S3 nanoparticles with controlled sizes and morphologies by adjusting the temperature and precursor concentrations. For instance, nanoparticles synthesized at low temperatures (25 °C) exhibit distinct worm-like structures, while higher temperatures (70 °C) lead to flower-like formations .
- Starch-Assisted Synthesis : Utilizing starch as a stabilizing agent results in enhanced stability and uniformity of Bi2S3 nanoparticles, which can improve their biological interactions .
- Morphological Variations :
Biological Activity
Bismuth sulfide exhibits several biological activities that make it a candidate for various applications, particularly in cancer therapy and antimicrobial treatments.
Anticancer Activity
Numerous studies have reported the potential of Bi2S3 nanoparticles as anticancer agents. Their mechanism often involves:
- Photothermal Therapy : Bi2S3 nanoparticles can convert light into heat effectively, which can be utilized to induce localized hyperthermia in tumor cells. This method enhances the cytotoxic effects on cancer cells while minimizing damage to surrounding healthy tissues .
- Reactive Oxygen Species (ROS) Production : Upon exposure to light, Bi2S3 generates ROS that can induce oxidative stress in cancer cells, leading to apoptosis. This property has been exploited in various in vitro studies demonstrating significant cytotoxicity against different cancer cell lines .
Antimicrobial Properties
Bi2S3 has also shown promising antimicrobial activity against a range of pathogens:
- Mechanism of Action : The antimicrobial effect is believed to be due to the disruption of bacterial cell membranes and the generation of ROS that can damage cellular components . Studies indicate that Bi2S3 nanoparticles can inhibit the growth of both Gram-positive and Gram-negative bacteria.
- Applications : These properties make Bi2S3 suitable for use in coatings for medical devices or as a component in wound dressings to prevent infections.
Case Studies
- Anticancer Efficacy :
- Antimicrobial Activity :
Data Tables
Property | Value/Observation |
---|---|
Particle Size | 15-40 nm |
Morphology | Worm-like, sponge-like, flower-like |
Anticancer Activity | Significant cytotoxicity against MCF-7 cells |
Antimicrobial Efficacy | Effective against S. aureus and E. coli |
Propiedades
IUPAC Name |
sulfanylidene(sulfanylidenebismuthanylsulfanyl)bismuthane | |
---|---|---|
Source | PubChem | |
URL | https://pubchem.ncbi.nlm.nih.gov | |
Description | Data deposited in or computed by PubChem | |
InChI |
InChI=1S/2Bi.3S | |
Source | PubChem | |
URL | https://pubchem.ncbi.nlm.nih.gov | |
Description | Data deposited in or computed by PubChem | |
InChI Key |
ZABYETDLLXARHZ-UHFFFAOYSA-N | |
Source | PubChem | |
URL | https://pubchem.ncbi.nlm.nih.gov | |
Description | Data deposited in or computed by PubChem | |
Canonical SMILES |
S=[Bi]S[Bi]=S | |
Source | PubChem | |
URL | https://pubchem.ncbi.nlm.nih.gov | |
Description | Data deposited in or computed by PubChem | |
Molecular Formula |
Bi2S3 | |
Source | PubChem | |
URL | https://pubchem.ncbi.nlm.nih.gov | |
Description | Data deposited in or computed by PubChem | |
Related CAS |
12048-34-9 (Parent), 7783-06-4 (Parent) | |
Record name | Bismuth sulfide | |
Source | ChemIDplus | |
URL | https://pubchem.ncbi.nlm.nih.gov/substance/?source=chemidplus&sourceid=0001345079 | |
Description | ChemIDplus is a free, web search system that provides access to the structure and nomenclature authority files used for the identification of chemical substances cited in National Library of Medicine (NLM) databases, including the TOXNET system. | |
Molecular Weight |
514.2 g/mol | |
Source | PubChem | |
URL | https://pubchem.ncbi.nlm.nih.gov | |
Description | Data deposited in or computed by PubChem | |
CAS No. |
1345-07-9 | |
Record name | Bismuth sulfide | |
Source | ChemIDplus | |
URL | https://pubchem.ncbi.nlm.nih.gov/substance/?source=chemidplus&sourceid=0001345079 | |
Description | ChemIDplus is a free, web search system that provides access to the structure and nomenclature authority files used for the identification of chemical substances cited in National Library of Medicine (NLM) databases, including the TOXNET system. | |
Record name | Bismuth sulfide (Bi2S3) | |
Source | EPA Chemicals under the TSCA | |
URL | https://www.epa.gov/chemicals-under-tsca | |
Description | EPA Chemicals under the Toxic Substances Control Act (TSCA) collection contains information on chemicals and their regulations under TSCA, including non-confidential content from the TSCA Chemical Substance Inventory and Chemical Data Reporting. | |
Descargo de responsabilidad e información sobre productos de investigación in vitro
Tenga en cuenta que todos los artículos e información de productos presentados en BenchChem están destinados únicamente con fines informativos. Los productos disponibles para la compra en BenchChem están diseñados específicamente para estudios in vitro, que se realizan fuera de organismos vivos. Los estudios in vitro, derivados del término latino "in vidrio", involucran experimentos realizados en entornos de laboratorio controlados utilizando células o tejidos. Es importante tener en cuenta que estos productos no se clasifican como medicamentos y no han recibido la aprobación de la FDA para la prevención, tratamiento o cura de ninguna condición médica, dolencia o enfermedad. Debemos enfatizar que cualquier forma de introducción corporal de estos productos en humanos o animales está estrictamente prohibida por ley. Es esencial adherirse a estas pautas para garantizar el cumplimiento de los estándares legales y éticos en la investigación y experimentación.