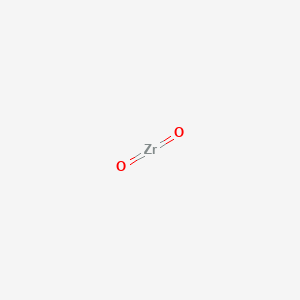
Zirconium dioxide
Descripción general
Descripción
Zirconium dioxide is a white crystalline oxide of zirconium . Its most naturally occurring form, with a monoclinic crystalline structure, is the mineral baddeleyite . A dopant stabilized cubic structured zirconia, cubic zirconia, is synthesized in various colors for use as a gemstone and a diamond simulant .
Synthesis Analysis
Zirconia is produced by calcining zirconium compounds, exploiting its high thermostability . Green synthesis of ZrO2 nanoparticles and their nanocomposites using compounds from bacteria, fungi, microalgae, and plants has been reviewed . Zirconium dioxide can be prepared from zirconium ores, the most abundant of which is zircon (ZrSiO4). The ore is usually purified via chlorination in the presence of carbon, followed by reduction to the metallic form, and finally, oxidation to yield pure ZrO2 .
Molecular Structure Analysis
Three phases of Zirconium dioxide are known: monoclinic below 1170 °C, tetragonal between 1170 °C and 2370 °C, and cubic above 2370 °C . A small percentage of the oxides of calcium or yttrium stabilize in the cubic phase . Unlike TiO2, which features six-coordinated titanium in all phases, monoclinic zirconia consists of seven-coordinated zirconium centers .
Chemical Reactions Analysis
Zirconia is chemically unreactive. It is slowly attacked by concentrated hydrofluoric acid and sulfuric acid. When heated with carbon, it converts to zirconium carbide. When heated with carbon in the presence of chlorine, it converts to zirconium (IV) chloride .
Physical And Chemical Properties Analysis
Zirconium dioxide is characterized by its high thermal resistivity, mechanical resistance, and abrasive properties . It has a high melting point of 2715 °C and a boiling point of 4300 °C . It is insoluble in water but soluble in HF and hot H2SO4 . It has a molar mass of 123.218 g/mol and a density of 5.68 g/cm3 .
Aplicaciones Científicas De Investigación
Photoluminescence and Structural Properties
Zirconium Oxide (ZrO2) thin films are produced using radio frequency magnetron sputtering method on glass substrate at various pressures . The effects of growth pressure on the characteristics of grown Zirconia nanostructures are investigated . ZrO2 thin films are considered potential materials for transparent electronic devices because of their high transmittance value .
Industrial Applications
Zirconium dioxide is used in various industries due to its physical and chemical properties . It is used in the automotive industry, electronics industry, energy and industrial ecology, equipment manufacturing and mechanical engineering . It is also used in the production of zirconium-based refractories, ceramics, enamels, glass, superhard materials .
Catalysts and Corrosion-Resistant Composites
Zirconium dioxide is utilized in several goods, including catalysts and corrosion-resistant composites, due to its superior chemical and physical benefits .
High Hardness and Strength Materials
Zirconium dioxide, possessing a number of specific properties, is widely used to obtain structural and functional materials with high hardness, strength, crack resistance, wear resistance, and chemical corrosion .
High Dielectric Constant Materials
Zirconium-based high-k gate dielectrics are prepared using many deposition processes such as atomic layer deposition (ALD), sol–gel process, chemical vapor deposition (CVD), sputtering, molecular beam epitaxy (MBE), electron beam evaporation (EBE), pulsed laser deposition (PLD) .
Oxygen Gas Sensors and Fuel Cells
Zirconium dioxide is preferred in many important applications such as oxygen gas sensors and as a component of solid oxide fuel cells due to its excellent properties .
Biological Ceramics and Memory Devices
Zirconium dioxide is used in biological ceramics and memory devices .
Jewelry Stone (Fianite)
Cubic modification of zirconium dioxide, stabilized by oxides of rare earth elements, is a jewelry stone (fianite) .
Mecanismo De Acción
Target of Action
Zirconium dioxide, also known as zirconia, is a white crystalline oxide of zirconium . It is primarily used in the ceramics industry due to its high thermal resistivity, mechanical resistance, and abrasive properties . Its most naturally occurring form, with a monoclinic crystalline structure, is the mineral baddeleyite .
Mode of Action
Zirconia is chemically unreactive. It is slowly attacked by concentrated hydrofluoric acid and sulfuric acid. When heated with carbon, it converts to zirconium carbide. When heated with carbon in the presence of chlorine, it converts to zirconium (IV) chloride . This conversion is the basis for the purification of zirconium metal .
Biochemical Pathways
Zirconia nanoparticles have been synthesized using compounds from bacteria, fungi, microalgae, and plants, which allow reducing, capping, chelating, and stabilizing during the transformation of zr4+ into zro2 nanoparticles .
Pharmacokinetics
Zirconia-based nanomaterials have been noted for their high stability and biocompatibility, which could potentially impact their bioavailability .
Result of Action
Zirconia nanoparticles have shown unique properties such as a nanoscale size of 5–50 nm, diverse morphologies, e.g., nanospheres, nanorods, and nanochains, and wide bandgap energy of 3.7–5.5 eV . Their high stability and biocompatibility make them suitable for various biomedical and environmental applications, such as pathogen and cancer inactivation, and pollutant removal .
Action Environment
The action of zirconium dioxide can be influenced by environmental factors. For instance, the synthesis of zirconia nanoparticles can be affected by the presence of bacteria, fungi, microalgae, and plants . Additionally, the presence of other elements or compounds, such as calcium or yttrium, can stabilize zirconia in the cubic phase .
Direcciones Futuras
Propiedades
IUPAC Name |
dioxozirconium | |
---|---|---|
Source | PubChem | |
URL | https://pubchem.ncbi.nlm.nih.gov | |
Description | Data deposited in or computed by PubChem | |
InChI |
InChI=1S/2O.Zr | |
Source | PubChem | |
URL | https://pubchem.ncbi.nlm.nih.gov | |
Description | Data deposited in or computed by PubChem | |
InChI Key |
MCMNRKCIXSYSNV-UHFFFAOYSA-N | |
Source | PubChem | |
URL | https://pubchem.ncbi.nlm.nih.gov | |
Description | Data deposited in or computed by PubChem | |
Canonical SMILES |
O=[Zr]=O | |
Source | PubChem | |
URL | https://pubchem.ncbi.nlm.nih.gov | |
Description | Data deposited in or computed by PubChem | |
Molecular Formula |
ZrO2, O2Zr | |
Record name | Zirconium(IV) oxide | |
Source | Wikipedia | |
URL | https://en.wikipedia.org/wiki/Zirconium(IV)_oxide | |
Description | Chemical information link to Wikipedia. | |
Record name | zirconium dioxide | |
Source | Wikipedia | |
URL | https://en.wikipedia.org/wiki/Zirconium_dioxide | |
Description | Chemical information link to Wikipedia. | |
Source | PubChem | |
URL | https://pubchem.ncbi.nlm.nih.gov | |
Description | Data deposited in or computed by PubChem | |
DSSTOX Substance ID |
DTXSID301315847 | |
Record name | Baddeleyite (ZrO2) | |
Source | EPA DSSTox | |
URL | https://comptox.epa.gov/dashboard/DTXSID301315847 | |
Description | DSSTox provides a high quality public chemistry resource for supporting improved predictive toxicology. | |
Molecular Weight |
123.22 g/mol | |
Source | PubChem | |
URL | https://pubchem.ncbi.nlm.nih.gov | |
Description | Data deposited in or computed by PubChem | |
Product Name |
Zirconium dioxide | |
CAS RN |
12036-23-6, 1314-23-4 | |
Record name | Baddeleyite (ZrO2) | |
Source | CAS Common Chemistry | |
URL | https://commonchemistry.cas.org/detail?cas_rn=12036-23-6 | |
Description | CAS Common Chemistry is an open community resource for accessing chemical information. Nearly 500,000 chemical substances from CAS REGISTRY cover areas of community interest, including common and frequently regulated chemicals, and those relevant to high school and undergraduate chemistry classes. This chemical information, curated by our expert scientists, is provided in alignment with our mission as a division of the American Chemical Society. | |
Explanation | The data from CAS Common Chemistry is provided under a CC-BY-NC 4.0 license, unless otherwise stated. | |
Record name | Zirconium dioxide | |
Source | CAS Common Chemistry | |
URL | https://commonchemistry.cas.org/detail?cas_rn=1314-23-4 | |
Description | CAS Common Chemistry is an open community resource for accessing chemical information. Nearly 500,000 chemical substances from CAS REGISTRY cover areas of community interest, including common and frequently regulated chemicals, and those relevant to high school and undergraduate chemistry classes. This chemical information, curated by our expert scientists, is provided in alignment with our mission as a division of the American Chemical Society. | |
Explanation | The data from CAS Common Chemistry is provided under a CC-BY-NC 4.0 license, unless otherwise stated. | |
Record name | Zirconium dioxide | |
Source | ChemIDplus | |
URL | https://pubchem.ncbi.nlm.nih.gov/substance/?source=chemidplus&sourceid=0001314234 | |
Description | ChemIDplus is a free, web search system that provides access to the structure and nomenclature authority files used for the identification of chemical substances cited in National Library of Medicine (NLM) databases, including the TOXNET system. | |
Record name | Baddeleyite (ZrO2) | |
Source | ChemIDplus | |
URL | https://pubchem.ncbi.nlm.nih.gov/substance/?source=chemidplus&sourceid=0012036236 | |
Description | ChemIDplus is a free, web search system that provides access to the structure and nomenclature authority files used for the identification of chemical substances cited in National Library of Medicine (NLM) databases, including the TOXNET system. | |
Record name | Zirconium dioxide | |
Source | DrugBank | |
URL | https://www.drugbank.ca/drugs/DB16552 | |
Description | The DrugBank database is a unique bioinformatics and cheminformatics resource that combines detailed drug (i.e. chemical, pharmacological and pharmaceutical) data with comprehensive drug target (i.e. sequence, structure, and pathway) information. | |
Explanation | Creative Common's Attribution-NonCommercial 4.0 International License (http://creativecommons.org/licenses/by-nc/4.0/legalcode) | |
Record name | Zirconium dioxide | |
Source | DTP/NCI | |
URL | https://dtp.cancer.gov/dtpstandard/servlet/dwindex?searchtype=NSC&outputformat=html&searchlist=12958 | |
Description | The NCI Development Therapeutics Program (DTP) provides services and resources to the academic and private-sector research communities worldwide to facilitate the discovery and development of new cancer therapeutic agents. | |
Explanation | Unless otherwise indicated, all text within NCI products is free of copyright and may be reused without our permission. Credit the National Cancer Institute as the source. | |
Record name | Baddeleyite (ZrO2) | |
Source | EPA DSSTox | |
URL | https://comptox.epa.gov/dashboard/DTXSID301315847 | |
Description | DSSTox provides a high quality public chemistry resource for supporting improved predictive toxicology. | |
Record name | Zirconium dioxide | |
Source | European Chemicals Agency (ECHA) | |
URL | https://echa.europa.eu/substance-information/-/substanceinfo/100.013.844 | |
Description | The European Chemicals Agency (ECHA) is an agency of the European Union which is the driving force among regulatory authorities in implementing the EU's groundbreaking chemicals legislation for the benefit of human health and the environment as well as for innovation and competitiveness. | |
Explanation | Use of the information, documents and data from the ECHA website is subject to the terms and conditions of this Legal Notice, and subject to other binding limitations provided for under applicable law, the information, documents and data made available on the ECHA website may be reproduced, distributed and/or used, totally or in part, for non-commercial purposes provided that ECHA is acknowledged as the source: "Source: European Chemicals Agency, http://echa.europa.eu/". Such acknowledgement must be included in each copy of the material. ECHA permits and encourages organisations and individuals to create links to the ECHA website under the following cumulative conditions: Links can only be made to webpages that provide a link to the Legal Notice page. | |
Record name | Baddeleyite (ZrO2) | |
Source | European Chemicals Agency (ECHA) | |
URL | https://echa.europa.eu/substance-information/-/substanceinfo/100.031.663 | |
Description | The European Chemicals Agency (ECHA) is an agency of the European Union which is the driving force among regulatory authorities in implementing the EU's groundbreaking chemicals legislation for the benefit of human health and the environment as well as for innovation and competitiveness. | |
Explanation | Use of the information, documents and data from the ECHA website is subject to the terms and conditions of this Legal Notice, and subject to other binding limitations provided for under applicable law, the information, documents and data made available on the ECHA website may be reproduced, distributed and/or used, totally or in part, for non-commercial purposes provided that ECHA is acknowledged as the source: "Source: European Chemicals Agency, http://echa.europa.eu/". Such acknowledgement must be included in each copy of the material. ECHA permits and encourages organisations and individuals to create links to the ECHA website under the following cumulative conditions: Links can only be made to webpages that provide a link to the Legal Notice page. | |
Q & A
Q1: What makes zirconia a suitable material for dental restorations?
A1: Zirconia possesses excellent biocompatibility [], [], high flexural strength [], and favorable esthetics [], making it suitable for dental applications.
Q2: How does the sintering process affect zirconia's properties?
A2: The sintering process can influence zirconia's phase composition and mechanical properties. For instance, high-speed sintering can alter the microstructure and mechanical characteristics compared to traditional sintering methods [].
Q3: Is zirconia susceptible to degradation in the oral environment?
A3: While zirconia exhibits excellent biocompatibility, some studies have reported a low incidence of aging and degradation of zirconia in vivo, highlighting the need for further investigation [].
Q4: How does zirconia compare to titanium for dental implants in terms of bacterial adhesion?
A4: Zirconia exhibits significantly lower adhesion of periodontopathogenic and cariogenic bacteria compared to traditional titanium alloys, suggesting a potential advantage in reducing the risk of infections [].
Q5: What is the chemical formula and molecular weight of zirconia?
A5: The chemical formula of zirconia is ZrO2, and its molecular weight is 123.22 g/mol.
Q6: What are the different crystalline phases of zirconia?
A6: Zirconia exists in three main polymorphs: monoclinic, tetragonal, and cubic []. The tetragonal phase is often stabilized for dental applications due to its superior mechanical properties [].
Q7: Can zirconia be used as a catalyst?
A7: Yes, zirconia can act as a catalyst, particularly in its sulfated form (SO4 2− /ZrO2), demonstrating activity in selective catalytic reduction (SCR) of nitrogen oxides with hydrocarbons [].
Q8: How does the addition of metal oxides influence zirconia's catalytic properties?
A8: Adding metal oxides like cobalt, iron, and manganese to sulfated zirconia can enhance its catalytic activity in SCR reactions [].
Q9: How does the presence of water vapor and sulfur dioxide affect zirconia-based catalysts?
A9: Unlike many zeolite and oxide catalysts, sulfated zirconia systems demonstrate low sensitivity to water vapor and sulfur dioxide, making them suitable for SCR processes in challenging environments [].
Q10: How does the position of yttrium atoms in the zirconia lattice affect its properties?
A10: Theoretical studies using Density Functional Theory (DFT) reveal that the position of yttrium atoms in the zirconia crystal lattice influences its surface charge density and adsorption properties [].
Q11: Does the presence of yttrium impact the hydration behavior of zirconia?
A11: Yes, DFT calculations indicate that the surface charge density of the 001 surface of a tetragonal zirconia plate increases with the degree of hydration, especially in the presence of yttrium [].
Q12: What analytical techniques are employed to study the bonding interface of zirconia restorations?
A12: Non-destructive techniques like Focused Ion Beam (FIB) technology combined with Scanning Electron Microscopy (SEM), Energy Dispersive X-ray (EDX) mapping, and conventional microscopy are used to analyze the bonding interface of zirconia restorations [].
Q13: What methods are used to study the mechanical properties of zirconia composites?
A13: Mechanical properties such as bending strength, modulus of elasticity, and fracture resistance are typically evaluated using tests like the three-point bending flexion test [], lateral unconfined compressive test [], and chewing simulations [].
Q14: How do composite resins reinforced with zirconia and titania compare to unmodified resins for provisional restorations?
A14: The addition of titania and zirconia mixtures to acrylic resins can enhance mechanical properties like bending strength and toughness, improving their suitability for provisional fixed restorations [].
Q15: What types of dental restorations can be fabricated using zirconia?
A15: Zirconia is used for various restorations, including full and partial coverage crowns, fixed partial dentures, veneers, posts and cores, implant abutments, and implants [], [], [].
Q16: What are the advantages of using zirconia in implant dentistry?
A16: Besides its biocompatibility and strength, zirconia's tooth-like color makes it aesthetically appealing for implant-supported restorations, especially in esthetically demanding areas [].
Q17: What are "root analogue" zirconia implants?
A17: Root analogue zirconia implants are designed to replicate the natural anatomy of extracted teeth, offering a "socket friendly" approach to immediate implantation [], [].
Q18: How does the clinical performance of zirconia implants compare to titanium implants?
A18: While zirconia implants demonstrate promising clinical outcomes, including good osseointegration [], long-term clinical studies are needed to definitively compare their performance with titanium implants [].
Q19: What is Rapid Layer Technology (RLT), and how is it used in zirconia restorations?
A19: RLT utilizes CAD/CAM technology to fabricate veneer layers that are adhesively bonded to zirconia frameworks, eliminating the need for firing steps and potentially increasing restoration lifetime [].
Q20: What types of cements are used for fixing zirconia restorations?
A20: Various cements are used for zirconia restorations, and the choice often depends on factors like bond strength, handling properties, and clinical situation [].
Q21: How can the bond strength between zirconia and dental cements be improved?
A21: Surface treatments, such as air-abrasion, etching with hydrofluoric acid, and the use of primers containing silane, can significantly enhance the bond strength between zirconia and dental cements [], [].
Q22: What is the influence of the abutment material on the optical outcome of zirconia crowns?
A22: Zirconia abutments demonstrate the lowest color difference (ΔE) values compared to titanium and anodized titanium abutments, indicating better aesthetic outcomes [].
Q23: Does the thickness of peri-implant soft tissue affect the color perception of zirconia restorations?
A23: While soft tissue thickness can impact the overall aesthetics, studies show that it does not significantly affect the color difference (ΔE) values of zirconia restorations at the peri-implant soft tissue level [].
Descargo de responsabilidad e información sobre productos de investigación in vitro
Tenga en cuenta que todos los artículos e información de productos presentados en BenchChem están destinados únicamente con fines informativos. Los productos disponibles para la compra en BenchChem están diseñados específicamente para estudios in vitro, que se realizan fuera de organismos vivos. Los estudios in vitro, derivados del término latino "in vidrio", involucran experimentos realizados en entornos de laboratorio controlados utilizando células o tejidos. Es importante tener en cuenta que estos productos no se clasifican como medicamentos y no han recibido la aprobación de la FDA para la prevención, tratamiento o cura de ninguna condición médica, dolencia o enfermedad. Debemos enfatizar que cualquier forma de introducción corporal de estos productos en humanos o animales está estrictamente prohibida por ley. Es esencial adherirse a estas pautas para garantizar el cumplimiento de los estándares legales y éticos en la investigación y experimentación.