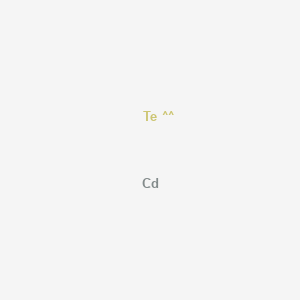
Teluro de cadmio
Descripción general
Descripción
Cadmium telluride is a stable crystalline compound formed from cadmium and tellurium. It is primarily used as a semiconducting material in cadmium telluride photovoltaics and as an infrared optical window . This compound is known for its excellent photovoltaic properties, making it a popular choice for thin-film solar cells .
Aplicaciones Científicas De Investigación
Cadmium telluride has a wide range of scientific research applications:
Mecanismo De Acción
Target of Action
Cadmium Telluride (CdTe) is a compound formed from Cadmium (Cd) and Tellurium (Te). It primarily targets the absorption of light, which is why it plays a critical role in CdTe solar cells . It is also used as a radiation detector due to its large atomic number, high resistivity at room temperature , and its extraordinary advantages like low weight, high mechanical hardness, and flexibility .
Mode of Action
CdTe operates as a semiconductor, absorbing and converting sunlight into electricity . In a solar cell, the CdTe absorber is attached to other materials, allowing electric current to flow through the absorber layer into the metal contacts and be collected as sustainable electricity . Modern cells often use Cadmium Selenium Tellurium (CdSeTe) in conjunction with CdTe to improve light absorption .
Biochemical Pathways
CdTe’s primary biochemical pathway involves the absorption of light and the subsequent generation of electric current. It is also known to induce oxidative stress—a pivotal mechanism behind its toxicity . This disruption of the balance between oxidants and antioxidants leads to cellular damage and apoptosis .
Pharmacokinetics
The toxicity of cadmium is an environmental concern during production and when the panels are disposed of .
Result of Action
The primary result of CdTe’s action is the conversion of sunlight into electricity, making it a key player in photovoltaic technology . Substantial cdte accumulation might result in organ injuries . It’s also worth noting that CdTe’s energy payback time of less than a year allows for faster carbon reductions without short-term energy deficits .
Action Environment
The action of CdTe is influenced by environmental factors. For instance, CdTe’s performance as a solar cell is dependent on the availability of sunlight. Additionally, the toxicity of cadmium is an environmental concern during production and when the panels are disposed of . The usage of rare materials may also become a limiting factor to the industrial scalability of CdTe technology in the mid-term future .
Análisis Bioquímico
Biochemical Properties
Cadmium telluride nanoparticles are fluorescent and may be used as quantum dots in imaging and diagnosis . The biochemical mechanisms of tellurium cytotoxicity are beginning to emerge .
Cellular Effects
It is known that the antibiotic properties of tellurite, a compound related to tellurium, have attracted renewed interest .
Molecular Mechanism
The close chemical relationship between tellurium and sulfur also transcends into in vitro and in vivo situations and provides new impetus for the development of enzyme inhibitors and redox modulators .
Métodos De Preparación
Synthetic Routes and Reaction Conditions: Cadmium telluride can be synthesized through various methods, including melt growth, vapor phase deposition, and solution growth. The melt growth method, such as the Bridgman-Stockbarger technique, is commonly used for producing large stoichiometric crystals . Vapor phase deposition involves the deposition of cadmium telluride vapor onto a substrate, while solution growth involves the precipitation of cadmium telluride from a solution containing cadmium and tellurium ions .
Industrial Production Methods: Industrial production of cadmium telluride involves the refinement of cadmium and tellurium, which are byproducts of zinc and copper mining, respectively . The refined materials are then used in processes such as vapor deposition to create cadmium telluride thin films for photovoltaic applications .
Análisis De Reacciones Químicas
Types of Reactions: Cadmium telluride undergoes various chemical reactions, including oxidation, reduction, and substitution.
Common Reagents and Conditions:
Major Products: The major products formed from these reactions include cadmium oxide, tellurium dioxide, elemental cadmium, and elemental tellurium .
Comparación Con Compuestos Similares
- Cadmium sulfide
- Cadmium selenide
- Zinc telluride
- Mercury cadmium telluride
Comparison: Cadmium telluride is unique due to its optimal band gap of 1.5 eV, which is ideal for photovoltaic applications . Compared to cadmium sulfide and cadmium selenide, cadmium telluride has a higher absorption coefficient, making it more efficient in converting sunlight into electricity . Zinc telluride and mercury cadmium telluride are also used in similar applications, but cadmium telluride remains the preferred choice for thin-film solar cells due to its cost-effectiveness and efficiency .
Actividad Biológica
Cadmium telluride (CdTe) is a compound that has garnered attention due to its applications in various fields, including photovoltaics and nanotechnology. However, its biological activity, particularly concerning toxicity, has raised significant concerns. This article explores the biological effects of cadmium telluride, focusing on its cytotoxicity, mechanisms of action, and implications for health and the environment.
Overview of Cadmium Telluride
Cadmium telluride is a semiconductor material used in solar cells and as quantum dots (QDs) in biomedical applications. While its properties make it valuable in technology, cadmium is a known toxic heavy metal that poses risks to human health and the environment.
Cytotoxicity of Cadmium Telluride Quantum Dots (CdTe-QDs)
Research has demonstrated that CdTe-QDs exhibit significant cytotoxic effects across various cell types. The following sections summarize key findings from studies investigating their biological activity.
Mitochondrial Toxicity
A study investigating the effects of CdTe-QDs on human hepatocellular carcinoma HepG2 cells revealed that these nanoparticles induce mitochondrial dysfunction. Key findings included:
- Mitochondrial Morphology Changes : CdTe-QDs caused enlargement and structural changes in mitochondria.
- Impaired Cellular Respiration : There was a notable disruption in mitochondrial membrane potential and decreased ATP synthesis.
- Increased Intracellular Calcium Levels : This elevation suggests a disturbance in calcium homeostasis, impacting cellular signaling pathways.
- Comparison with Cadmium Chloride : The toxic effects of CdTe-QDs were found to be similar or greater than those caused by cadmium chloride at equivalent concentrations, indicating that toxicity may not solely arise from cadmium release .
Liver Toxicity
In vivo studies involving mice have shown that exposure to CdTe-QDs leads to liver toxicity characterized by:
- Increased Lipid Peroxidation : Elevated levels of malondialdehyde (MDA), a marker for oxidative stress, were observed.
- Cytotoxicity in Hepatoma Cells : The cytotoxic effects were dose- and time-dependent, mediated by reactive oxygen species (ROS) generation and apoptosis induction.
- Serum Biochemical Changes : Parameters such as total protein, albumin, and liver enzymes (ALT, AST) were assessed to evaluate liver function post-exposure .
Mechanisms of Toxicity
The mechanisms underlying the toxicity of CdTe-QDs are multifaceted:
- Reactive Oxygen Species (ROS) Generation : CdTe-QDs induce oxidative stress through ROS production, leading to cellular damage.
- Programmed Cell Death : Activation of apoptotic pathways is noted in various cell types exposed to these nanoparticles.
- Impact on Cellular Signaling : Alterations in immune signaling responses have been documented in macrophages and epithelial cells exposed to CdTe-QDs .
Table 1: Summary of Key Findings on CdTe-QD Toxicity
Environmental Impact
Cadmium compounds, including CdTe, pose risks not only to human health but also to environmental ecosystems. Studies indicate that aquatic organisms are particularly sensitive to cadmium exposure, leading to detrimental effects on biodiversity and ecosystem health. Accumulation of CdTe-QDs in plant systems has also been documented, raising concerns about food safety and bioaccumulation .
Propiedades
IUPAC Name |
tellanylidenecadmium | |
---|---|---|
Source | PubChem | |
URL | https://pubchem.ncbi.nlm.nih.gov | |
Description | Data deposited in or computed by PubChem | |
InChI |
InChI=1S/Cd.Te | |
Source | PubChem | |
URL | https://pubchem.ncbi.nlm.nih.gov | |
Description | Data deposited in or computed by PubChem | |
InChI Key |
RPPBZEBXAAZZJH-UHFFFAOYSA-N | |
Source | PubChem | |
URL | https://pubchem.ncbi.nlm.nih.gov | |
Description | Data deposited in or computed by PubChem | |
Canonical SMILES |
[Cd]=[Te] | |
Source | PubChem | |
URL | https://pubchem.ncbi.nlm.nih.gov | |
Description | Data deposited in or computed by PubChem | |
Molecular Formula |
CdTe | |
Record name | cadmium telluride | |
Source | Wikipedia | |
URL | https://en.wikipedia.org/wiki/Cadmium_telluride | |
Description | Chemical information link to Wikipedia. | |
Source | PubChem | |
URL | https://pubchem.ncbi.nlm.nih.gov | |
Description | Data deposited in or computed by PubChem | |
DSSTOX Substance ID |
DTXSID0030950 | |
Record name | Cadmium telluride | |
Source | EPA DSSTox | |
URL | https://comptox.epa.gov/dashboard/DTXSID0030950 | |
Description | DSSTox provides a high quality public chemistry resource for supporting improved predictive toxicology. | |
Molecular Weight |
240.0 g/mol | |
Source | PubChem | |
URL | https://pubchem.ncbi.nlm.nih.gov | |
Description | Data deposited in or computed by PubChem | |
Physical Description |
Brownish-black solid; Oxidizes on long term contact with moist air; [Merck Index] Black odorless pieces; [MSDSonline] | |
Record name | Cadmium telluride | |
Source | Haz-Map, Information on Hazardous Chemicals and Occupational Diseases | |
URL | https://haz-map.com/Agents/8440 | |
Description | Haz-Map® is an occupational health database designed for health and safety professionals and for consumers seeking information about the adverse effects of workplace exposures to chemical and biological agents. | |
Explanation | Copyright (c) 2022 Haz-Map(R). All rights reserved. Unless otherwise indicated, all materials from Haz-Map are copyrighted by Haz-Map(R). No part of these materials, either text or image may be used for any purpose other than for personal use. Therefore, reproduction, modification, storage in a retrieval system or retransmission, in any form or by any means, electronic, mechanical or otherwise, for reasons other than personal use, is strictly prohibited without prior written permission. | |
CAS No. |
1306-25-8 | |
Record name | Cadmium telluride | |
Source | CAS Common Chemistry | |
URL | https://commonchemistry.cas.org/detail?cas_rn=1306-25-8 | |
Description | CAS Common Chemistry is an open community resource for accessing chemical information. Nearly 500,000 chemical substances from CAS REGISTRY cover areas of community interest, including common and frequently regulated chemicals, and those relevant to high school and undergraduate chemistry classes. This chemical information, curated by our expert scientists, is provided in alignment with our mission as a division of the American Chemical Society. | |
Explanation | The data from CAS Common Chemistry is provided under a CC-BY-NC 4.0 license, unless otherwise stated. | |
Record name | Cadmium telluride | |
Source | ChemIDplus | |
URL | https://pubchem.ncbi.nlm.nih.gov/substance/?source=chemidplus&sourceid=0001306258 | |
Description | ChemIDplus is a free, web search system that provides access to the structure and nomenclature authority files used for the identification of chemical substances cited in National Library of Medicine (NLM) databases, including the TOXNET system. | |
Record name | Cadmium telluride (CdTe) | |
Source | EPA Chemicals under the TSCA | |
URL | https://www.epa.gov/chemicals-under-tsca | |
Description | EPA Chemicals under the Toxic Substances Control Act (TSCA) collection contains information on chemicals and their regulations under TSCA, including non-confidential content from the TSCA Chemical Substance Inventory and Chemical Data Reporting. | |
Record name | Cadmium telluride | |
Source | EPA DSSTox | |
URL | https://comptox.epa.gov/dashboard/DTXSID0030950 | |
Description | DSSTox provides a high quality public chemistry resource for supporting improved predictive toxicology. | |
Record name | Cadmium telluride | |
Source | European Chemicals Agency (ECHA) | |
URL | https://echa.europa.eu/substance-information/-/substanceinfo/100.013.773 | |
Description | The European Chemicals Agency (ECHA) is an agency of the European Union which is the driving force among regulatory authorities in implementing the EU's groundbreaking chemicals legislation for the benefit of human health and the environment as well as for innovation and competitiveness. | |
Explanation | Use of the information, documents and data from the ECHA website is subject to the terms and conditions of this Legal Notice, and subject to other binding limitations provided for under applicable law, the information, documents and data made available on the ECHA website may be reproduced, distributed and/or used, totally or in part, for non-commercial purposes provided that ECHA is acknowledged as the source: "Source: European Chemicals Agency, http://echa.europa.eu/". Such acknowledgement must be included in each copy of the material. ECHA permits and encourages organisations and individuals to create links to the ECHA website under the following cumulative conditions: Links can only be made to webpages that provide a link to the Legal Notice page. | |
Record name | CADMIUM TELLURIDE | |
Source | FDA Global Substance Registration System (GSRS) | |
URL | https://gsrs.ncats.nih.gov/ginas/app/beta/substances/STG188WO13 | |
Description | The FDA Global Substance Registration System (GSRS) enables the efficient and accurate exchange of information on what substances are in regulated products. Instead of relying on names, which vary across regulatory domains, countries, and regions, the GSRS knowledge base makes it possible for substances to be defined by standardized, scientific descriptions. | |
Explanation | Unless otherwise noted, the contents of the FDA website (www.fda.gov), both text and graphics, are not copyrighted. They are in the public domain and may be republished, reprinted and otherwise used freely by anyone without the need to obtain permission from FDA. Credit to the U.S. Food and Drug Administration as the source is appreciated but not required. | |
Q1: What is the chemical formula and molecular weight of Cadmium Telluride?
A1: Cadmium Telluride has the chemical formula CdTe, with a molecular weight of 240.01 g/mol.
Q2: What are the key optical properties of Cadmium Telluride relevant to solar cell applications?
A2: Cadmium telluride possesses a near-optimal band gap [] of around 1.5 eV, making it highly efficient at absorbing sunlight and converting it into electricity. Its direct bandgap allows for efficient absorption of photons across a wide range of the solar spectrum. [, , ] Additionally, CdTe can be fabricated as a thin film, enabling cost-effective solar cell production. [, , ]
Q3: How does the resistivity of p-type Cadmium Telluride thin films get controlled during fabrication?
A3: The resistivity of p-type CdTe thin films can be controlled by two methods: (1) using a cadmium-deficient reaction mixture during deposition or (2) introducing a dopant into a near-stoichiometric reaction mixture. []
Q4: What is the impact of temperature on the efficiency of Cadmium Telluride solar cells compared to other materials?
A4: Cadmium telluride-based solar cells demonstrate superior temperature stability compared to other thin-film technologies like copper indium selenide (CuInSe2), amorphous silicon, and crystalline gallium arsenide (GaAs). A temperature increase of 50°C results in only a 1% efficiency reduction for CdTe solar cells, with a relative decrease rate of -0.14 rel.%/°C. This is significantly lower than GaAs (-0.16 rel.%/°C), amorphous silicon (-0.21 rel.%/°C), and CuInSe2 (-0.36 rel.%/°C). []
Q5: What role does "chloride treatment" play in enhancing the performance of Cadmium Telluride solar cells?
A5: Chloride treatment, involving the deposition of cadmium chloride (CdCl2) or magnesium chloride (MgCl2) followed by annealing, is crucial for achieving high-efficiency CdTe solar cells. This process facilitates: - Transformation of metastable hexagonal CdTe to the stable cubic modification. [] - Recrystallization leading to larger coherent scattering regions and reduced microstrain. [] - Passivation of grain boundaries, reducing recombination losses and improving carrier lifetime. [, ] - Enhanced doping efficiency and improved electrical properties. [, ]
Q6: Besides solar cells, what other applications utilize Cadmium Telluride's properties?
A6: Cadmium telluride finds applications in several other areas: - Radiation detectors: CdTe is used in X-ray detectors [, ], gamma-ray detectors [, ], and energy-discriminating X-ray cameras []. Its high atomic number and wide bandgap make it suitable for detecting high-energy photons. - Medical imaging: CdTe-based detectors are employed in dental panoramic radiography [] and spectroscopic breast imaging. [] - Optoelectronic devices: CdTe is explored for its potential in laser space communication modulators. []
Q7: What are the challenges associated with using Cadmium Telluride in high-temperature applications like infrared detectors?
A7: While Cadmium Telluride is an attractive material for infrared detectors, its performance at high operating temperatures can be limited by: - Increased dark current: As temperature rises, the intrinsic carrier concentration increases, leading to higher dark current and reduced detectivity. - Material degradation: Prolonged exposure to elevated temperatures can result in material degradation and performance deterioration.
Q8: How is Cadmium Telluride being modified for improved performance in specific applications?
A8: Researchers are exploring various strategies to enhance the performance of CdTe in different applications: - Doping: Controlled doping with elements like copper can modify electrical properties and enhance performance in solar cells and detectors. [, , ] - Nano-structuring: Incorporating nano-grating structures within CdTe thin films can improve light absorption and boost solar cell efficiency. [] - Heterostructures: Combining CdTe with other materials like Cadmium Sulfide (CdS) in heterostructure configurations improves efficiency and stability in solar cells. [, , ] - Surface treatments: Surface passivation techniques aim to reduce surface recombination losses and improve device performance. [, ]
Descargo de responsabilidad e información sobre productos de investigación in vitro
Tenga en cuenta que todos los artículos e información de productos presentados en BenchChem están destinados únicamente con fines informativos. Los productos disponibles para la compra en BenchChem están diseñados específicamente para estudios in vitro, que se realizan fuera de organismos vivos. Los estudios in vitro, derivados del término latino "in vidrio", involucran experimentos realizados en entornos de laboratorio controlados utilizando células o tejidos. Es importante tener en cuenta que estos productos no se clasifican como medicamentos y no han recibido la aprobación de la FDA para la prevención, tratamiento o cura de ninguna condición médica, dolencia o enfermedad. Debemos enfatizar que cualquier forma de introducción corporal de estos productos en humanos o animales está estrictamente prohibida por ley. Es esencial adherirse a estas pautas para garantizar el cumplimiento de los estándares legales y éticos en la investigación y experimentación.