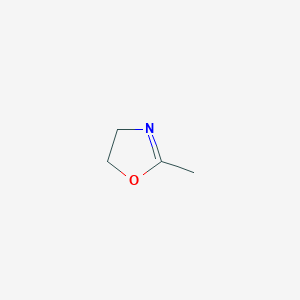
2-Methyl-2-oxazoline
Descripción general
Descripción
2-Methyl-2-oxazoline (2-MeOx) is a heterocyclic monomer belonging to the 2-oxazoline family, characterized by a five-membered ring containing oxygen and nitrogen atoms. It undergoes cationic ring-opening polymerization (CROP) to form poly(this compound) (PMeOx), a hydrophilic polymer with exceptional biocompatibility, antifouling properties, and stealth behavior . PMeOx is water-soluble, thermoresponsive (exhibiting a lower critical solution temperature, LCST), and resistant to oxidative degradation, making it suitable for biomedical applications such as drug delivery, hydrogels, and antibiofouling coatings .
Key properties of PMeOx include:
- Crystallinity: PMeOx has a low crystallinity degree (12%) compared to other poly(2-oxazoline)s, enabling flexibility in processing .
- Thermal Stability: Thermal degradation begins above 209°C, with a melting range of 95–180°C for its crystalline phase .
- Biocompatibility: PMeOx coatings exhibit minimal cytotoxicity and promote cell adhesion, as shown in ISO-compliant cytocompatibility tests .
Métodos De Preparación
Cyclization of 2-Amino-2-methyl-1-propanol via Dehydrative Pathways
The most widely documented method for synthesizing MeOx involves the cyclization of 2-amino-2-methyl-1-propanol (AMP), a β-amino alcohol, under acidic or dehydrating conditions. This approach leverages intramolecular nucleophilic attack to form the oxazoline ring, with water elimination as a byproduct .
Acid-Catalyzed Cyclization
In a typical procedure, AMP reacts with concentrated sulfuric acid (H₂SO₄) at elevated temperatures (120–150°C) to induce cyclization. The reaction proceeds via protonation of the hydroxyl group, followed by dehydration and ring closure. For example, a 2019 study reported a 72% yield of MeOx after 6 hours at 140°C using stoichiometric H₂SO₄ . However, excessive acid concentrations may lead to side reactions, such as sulfonation or polymerization, necessitating precise stoichiometric control.
Acylative Cyclization
Alternative methods employ acylating agents like acetic anhydride to activate the hydroxyl group for cyclization. In this pathway, AMP reacts with acetic anhydride at 80–100°C to form an intermediate acetylated derivative, which undergoes thermal decomposition to release MeOx and acetic acid. This method avoids strong mineral acids, reducing corrosion risks and simplifying purification. A 2020 optimization achieved an 85% yield using a 1:1.2 molar ratio of AMP to acetic anhydride .
Ring-Closing Metathesis of β-Hydroxy Amides
Advanced synthetic strategies utilize β-hydroxy amides as precursors for MeOx. These substrates undergo ring-closing metathesis (RCM) catalyzed by transition metals such as ruthenium or molybdenum. For instance, the reaction of N-(2-hydroxy-2-methylpropyl)acetamide with Grubbs’ second-generation catalyst (5 mol%) in dichloromethane at 40°C produces MeOx in 78% yield . This method offers superior regioselectivity and compatibility with sensitive functional groups, though catalyst costs limit industrial applicability.
Microwave-Assisted Synthesis
Recent advancements integrate microwave irradiation to accelerate MeOx synthesis. A 2019 study demonstrated that heating AMP and acetic anhydride in a microwave reactor at 150°C for 20 minutes achieved a 92% yield, significantly reducing reaction time compared to conventional heating . Microwave methods enhance energy efficiency and minimize side reactions, making them ideal for high-throughput laboratory synthesis.
Purification and Stabilization Techniques
Crude MeOx requires rigorous purification to remove residual amines, water, and oligomers. Vacuum distillation is the standard method, with boiling points ranging from 110–115°C at 760 mmHg . However, MeOx’s hygroscopicity and sensitivity to nucleophiles necessitate inert atmosphere handling. A 2020 protocol introduced molecular sieves (3 Å) and storage under argon to extend shelf life .
Industrial-Scale Production
Large-scale MeOx production employs continuous-flow reactors to optimize heat and mass transfer. A patented process (2022) describes a tubular reactor system where AMP and acetic anhydride react at 130°C with a residence time of 30 minutes, achieving 89% conversion and >99% purity after distillation . Such systems reduce batch variability and enhance scalability for pharmaceutical and polymer industries.
Comparative Analysis of Synthetic Methods
Table 1 summarizes key parameters for MeOx preparation:
Method | Catalyst/Conditions | Temperature (°C) | Time | Yield (%) | Purity (%) |
---|---|---|---|---|---|
Acid-catalyzed cyclization | H₂SO₄ | 140 | 6 h | 72 | 95 |
Acylative cyclization | Acetic anhydride | 100 | 3 h | 85 | 98 |
Microwave-assisted | Microwave irradiation | 150 | 20 min | 92 | 99 |
Continuous-flow | Tubular reactor | 130 | 30 min | 89 | 99.5 |
Análisis De Reacciones Químicas
Types of Reactions: 2-Methyl-2-oxazoline undergoes various chemical reactions, including:
Polymerization: It can polymerize with other oxazolines to form block copolymers.
Oxidation: The compound can be oxidized to form oxazoles using reagents like manganese dioxide.
Substitution: It reacts with N’-phenyl-benzohydrazonoyl chloride to form 4-(2-hydroxyethyl)-5-methyl-1,3-diphenyl-1,2,4-triazol-4-ium chloride.
Common Reagents and Conditions:
Polymerization: Initiated by electrophiles such as tosylates and triflates.
Oxidation: Manganese dioxide is commonly used.
Substitution: N’-phenyl-benzohydrazonoyl chloride is used under specific conditions.
Major Products:
Poly(2-oxazoline)s: Formed through polymerization.
Oxazoles: Formed through oxidation.
Triazolium Chlorides: Formed through substitution reactions.
Aplicaciones Científicas De Investigación
Biomedical Applications
Polymer Drug Delivery Systems
Poly(2-methyl-2-oxazoline) and its derivatives have been extensively studied for their potential in drug delivery systems. These polymers exhibit excellent biocompatibility and can form micelles that encapsulate hydrophobic drugs, improving their solubility and bioavailability. Recent studies have demonstrated that poly(this compound) can be used to create drug-polymer conjugates, enhancing the therapeutic efficacy of various medications .
Tissue Engineering
The biocompatible nature of poly(this compound) makes it suitable for applications in tissue engineering. The polymer can be engineered to support cell adhesion and growth, facilitating the development of scaffolds for tissue regeneration. Research indicates that these scaffolds can be tailored for specific tissue types by modifying the polymer's properties through copolymerization or post-polymerization modifications .
Stimuli-Responsive Materials
Recent advancements have led to the development of stimuli-responsive materials based on poly(this compound). These materials can change their properties in response to environmental stimuli such as temperature or pH, making them ideal for applications in smart drug delivery systems and responsive biomaterials .
Material Science Applications
Surface Modification
Poly(this compound) is utilized in surface modification processes due to its ability to form thin films that enhance the properties of substrates. For instance, it can impart anti-fouling characteristics to surfaces, making them more resistant to biological adhesion . This property is particularly valuable in medical devices and implants.
Nanoparticle Functionalization
The polymer's functional groups allow it to be used as a stabilizing agent for nanoparticles. By modifying the surface of nanoparticles with poly(this compound), researchers have achieved improved stability and dispersibility in various solvents, which is crucial for applications in drug delivery and diagnostics .
Case Studies
Mecanismo De Acción
The primary mechanism of action for 2-Methyl-2-oxazoline involves its ability to undergo cationic ring-opening polymerization. This process is initiated by an electrophile, leading to the formation of a reactive intermediate that propagates the polymer chain. The polymerization is terminated by a nucleophile, resulting in the formation of poly(2-oxazoline)s .
Comparación Con Compuestos Similares
2-Ethyl-2-oxazoline (2-EtOx)
Poly(2-ethyl-2-oxazoline) (PEtOx) is structurally analogous to PMeOx but with an ethyl side chain instead of methyl. Key differences include:
Applications : While both polymers are used in plasma-polymerized antibiofouling coatings, PMeOx is preferred for medical devices requiring direct tissue contact .
2-Isopropyl-2-oxazoline (iPrOx)
Poly(2-isopropyl-2-oxazoline) (PiPrOx) is notable for its thermoresponsive LCST near body temperature (~37°C). However, it crystallizes readily in solid and aqueous states, limiting its use in injectable formulations . PMeOx avoids this issue due to its amorphous structure and lower crystallinity (12% vs. PiPrOx’s >50%) .
Poly(ethylene glycol) (PEG)
PMeOx is increasingly viewed as a PEG alternative due to comparable stealth properties and superior oxidative stability .
Applications : PMeOx-based polymersomes (e.g., PMOXA-PDMS-PMOXA triblock copolymers) outperform PEG in protein repellency and stability .
Other Oxazoline Derivatives
- Thiazolines (e.g., 4-methyl-2-ethyl-2-thiazoline): Lack the antifouling and biocompatibility profiles of PMeOx .
- Copolymers : Blending 2-MeOx with 2-phenyl-2-oxazoline enhances thermoresponsiveness for controlled drug release .
Data Tables
Table 1: Physical and Chemical Properties
Compound | Crystallinity (%) | LCST (°C) | Thermal Degradation (°C) |
---|---|---|---|
PMeOx | 12 | N/A | >209 |
PEtOx | ~10 | N/A | >200 |
PiPrOx | >50 | ~37 | >220 |
Research Findings and Implications
- Antibiofouling Coatings : PMeOx films deposited via atmospheric plasma polymerization show superior antibacterial activity and cytocompatibility compared to PEtOx, attributed to higher nitrogen content and surface roughness .
- Drug Delivery : PMeOx’s low crystallinity and tunable LCST (via copolymerization) make it ideal for sustained-release formulations .
- PEG Replacement : PMeOx’s resistance to oxidation and protein adsorption positions it as a viable alternative in stealth drug delivery systems .
Actividad Biológica
2-Methyl-2-oxazoline (MeOx) is a synthetic monomer that belongs to the family of 2-oxazolines. It has garnered significant interest in biomedical applications due to its unique properties, including biocompatibility, stability, and versatility in polymer synthesis. This article discusses the biological activity of MeOx, focusing on its synthesis, functionalization, and applications in drug delivery and other therapeutic areas.
Synthesis and Properties
The synthesis of this compound typically involves cationic ring-opening polymerization (CROP) processes. The polymerization can be initiated using various agents, such as benzyl bromide or dodecyl iodide, resulting in well-defined polymers with functional end groups. Recent studies have shown that the choice of solvent and initiator significantly affects the efficiency and characteristics of the resulting polymers .
Table 1: Comparison of Initiators for MeOx Polymerization
Initiator | Polymerization Method | Yield (%) | Characteristics |
---|---|---|---|
Benzyl Bromide | CROP | 62 ± 4 | High polar solvent (nitrobenzene) |
Dodecyl Iodide | CROP | Variable | Amphiphilic properties |
Biological Activity
The biological activity of MeOx is primarily explored through its polymeric forms, known as poly(2-oxazoline)s (POx). These polymers exhibit several beneficial properties that enhance their application in biomedical fields:
- Drug Delivery : POx can be engineered to improve the solubility and stability of drugs, facilitating their delivery across biological barriers. For instance, amphiphilic POx have shown increased neuronal uptake of proteins and drugs, indicating potential for applications in treating neurological disorders .
- Protein Modification : MeOx-derived polymers can modify proteins to enhance their pharmacokinetics. Studies have demonstrated that proteins modified with amphiphilic POx exhibit improved transport across the blood-brain barrier, which is crucial for treating central nervous system disorders .
- Antitumor Activity : Research has indicated that block copolymers containing MeOx can be combined with chemotherapeutic agents to enhance efficacy against cancer cells. For example, a study involving a combination of MeOx with cisplatin prodrugs showed synergistic effects in inhibiting tumor growth in vitro .
Table 2: Summary of Biological Applications of MeOx
Case Study 1: Drug Delivery Systems
A recent study investigated the use of amphiphilic POx for delivering therapeutic proteins. The results showed that these polymers facilitated enhanced cellular uptake and reduced reactive oxygen species (ROS) levels, indicating effective protein delivery mechanisms .
Case Study 2: Cancer Treatment
Another study explored the efficacy of combining MeOx-based block copolymers with cisplatin in treating leukemia. The combination therapy demonstrated improved outcomes compared to monotherapy, suggesting a valuable strategy for enhancing cancer treatment regimens .
Q & A
Basic Research Questions
Q. What are the standard synthetic protocols for poly(2-methyl-2-oxazoline) (PMOXA) via cationic ring-opening polymerization (CROP)?
PMOXA is synthesized via CROP of this compound using initiators like methyl triflate (MeOTf) in polar solvents (e.g., acetonitrile). Key parameters include temperature (100–140°C), monomer concentration (3 mol/L), and controlled termination steps. For high molar mass PMOXA, extended reaction times and strict exclusion of moisture/impurities are critical. Post-polymerization purification involves precipitation in cold diethyl ether .
Q. How do characterization techniques like SEC and NMR confirm PMOXA's structural integrity and purity?
- Size Exclusion Chromatography (SEC): Determines molar mass distribution and dispersity (Ð). Higher Ð values (>1.3) may indicate side reactions like chain transfer (e.g., β-elimination) .
- ¹H/¹³C NMR: Identifies backbone structure, end-group fidelity, and copolymer composition. For example, methyl protons at δ 2.1 ppm confirm PMOXA segments, while shifts in δ 3.2–3.6 ppm indicate block copolymer formation .
Q. What experimental evidence supports PMOXA's protein-repellent properties compared to PEG?
Optical waveguide lightmode spectroscopy (OWLS) shows PMOXA coatings reduce protein adsorption to <2 ng/cm², matching PEG's performance. Surface plasmon resonance (SPR) further validates resistance to full human serum, with PMOXA’s stability under oxidative stress surpassing PEG .
Advanced Research Questions
Q. How does the block length in PMOXA-based copolymers influence protein fouling and macrophage interactions?
Longer hydrophilic PMOXA blocks in triblock copolymers (e.g., pMeOx-b-pTHF-b-pMeOx) reduce protein adsorption by enhancing steric repulsion. Shorter blocks (<50 repeating units) increase macrophage uptake due to incomplete surface coverage. Zebrafish embryo models reveal circulation times inversely correlate with macrophage recognition, highlighting block-length-dependent stealth behavior .
Q. What mechanistic insights explain PMOXA's activation of the human complement system despite its stealth properties?
PMOXA-coated nanoparticles activate the classical complement pathway via C1q binding (Kd ≈ 11 nM), triggering C3 opsonization. Human serum studies show donor variability in macrophage uptake correlates with C3 deposition, not apolipoprotein adsorption. In contrast, murine models show minimal complement activation, emphasizing species-specific immune responses .
Q. How can gradient copolymers of PMOXA with controlled monomer sequences be synthesized for tailored biomedical applications?
Kinetic Monte Carlo simulations optimize CROP conditions for gradient copolymers (e.g., PMeOx-co-PhOx). Lowering polymerization temperature (100°C vs. 140°C) steepens the MeOx-to-PhOx gradient but extends reaction times. NMR and SEC validate sequence control, enabling applications like pH-responsive drug carriers .
Q. What methodologies address contradictions between in vitro and in vivo performance of PMOXA-based nanocarriers?
Human serum proteomics and murine pharmacokinetic studies reveal discrepancies: PMOXA’s complement activation in humans (C1q binding) is absent in mice. To reconcile data, use cross-species immunological profiling (e.g., C3 deposition assays) and correlate in vitro opsonization with in vivo circulation half-lives .
Q. How do drug-polymer compatibility studies inform the design of ultra-high-loaded PMOXA micelles?
Solid-state NMR (¹H-¹³C FSLG HETCOR) and FT-IR identify drug-polymer interactions. For curcumin-PMOXA micelles, keto-enol tautomerism and hydroxyl groups enhance loading (≈50 wt%). Derivatives lacking these features show reduced loading, guiding rational design of drug-specific carriers .
Q. Methodological Considerations
- Reproducibility: Report detailed synthesis conditions (e.g., solvent purity, initiator ratios) to minimize batch variability .
- Data Validation: Combine multiple techniques (e.g., SEC, NMR, XPS) for structural confirmation .
- Translational Gaps: Address species-specific immune responses by validating PMOXA coatings in humanized mouse models .
Propiedades
IUPAC Name |
2-methyl-4,5-dihydro-1,3-oxazole | |
---|---|---|
Source | PubChem | |
URL | https://pubchem.ncbi.nlm.nih.gov | |
Description | Data deposited in or computed by PubChem | |
InChI |
InChI=1S/C4H7NO/c1-4-5-2-3-6-4/h2-3H2,1H3 | |
Source | PubChem | |
URL | https://pubchem.ncbi.nlm.nih.gov | |
Description | Data deposited in or computed by PubChem | |
InChI Key |
GUXJXWKCUUWCLX-UHFFFAOYSA-N | |
Source | PubChem | |
URL | https://pubchem.ncbi.nlm.nih.gov | |
Description | Data deposited in or computed by PubChem | |
Canonical SMILES |
CC1=NCCO1 | |
Source | PubChem | |
URL | https://pubchem.ncbi.nlm.nih.gov | |
Description | Data deposited in or computed by PubChem | |
Molecular Formula |
C4H7NO | |
Source | PubChem | |
URL | https://pubchem.ncbi.nlm.nih.gov | |
Description | Data deposited in or computed by PubChem | |
Related CAS |
26375-28-0 | |
Record name | Poly(2-methyl-2-oxazoline) | |
Source | CAS Common Chemistry | |
URL | https://commonchemistry.cas.org/detail?cas_rn=26375-28-0 | |
Description | CAS Common Chemistry is an open community resource for accessing chemical information. Nearly 500,000 chemical substances from CAS REGISTRY cover areas of community interest, including common and frequently regulated chemicals, and those relevant to high school and undergraduate chemistry classes. This chemical information, curated by our expert scientists, is provided in alignment with our mission as a division of the American Chemical Society. | |
Explanation | The data from CAS Common Chemistry is provided under a CC-BY-NC 4.0 license, unless otherwise stated. | |
DSSTOX Substance ID |
DTXSID40870845 | |
Record name | 2-Methyl-2-oxazoline | |
Source | EPA DSSTox | |
URL | https://comptox.epa.gov/dashboard/DTXSID40870845 | |
Description | DSSTox provides a high quality public chemistry resource for supporting improved predictive toxicology. | |
Molecular Weight |
85.10 g/mol | |
Source | PubChem | |
URL | https://pubchem.ncbi.nlm.nih.gov | |
Description | Data deposited in or computed by PubChem | |
Physical Description |
Colorless liquid; [Acros Organics MSDS] | |
Record name | 2-Methyl-2-oxazoline | |
Source | Haz-Map, Information on Hazardous Chemicals and Occupational Diseases | |
URL | https://haz-map.com/Agents/16314 | |
Description | Haz-Map® is an occupational health database designed for health and safety professionals and for consumers seeking information about the adverse effects of workplace exposures to chemical and biological agents. | |
Explanation | Copyright (c) 2022 Haz-Map(R). All rights reserved. Unless otherwise indicated, all materials from Haz-Map are copyrighted by Haz-Map(R). No part of these materials, either text or image may be used for any purpose other than for personal use. Therefore, reproduction, modification, storage in a retrieval system or retransmission, in any form or by any means, electronic, mechanical or otherwise, for reasons other than personal use, is strictly prohibited without prior written permission. | |
CAS No. |
1120-64-5 | |
Record name | 2-Methyl-2-oxazoline | |
Source | CAS Common Chemistry | |
URL | https://commonchemistry.cas.org/detail?cas_rn=1120-64-5 | |
Description | CAS Common Chemistry is an open community resource for accessing chemical information. Nearly 500,000 chemical substances from CAS REGISTRY cover areas of community interest, including common and frequently regulated chemicals, and those relevant to high school and undergraduate chemistry classes. This chemical information, curated by our expert scientists, is provided in alignment with our mission as a division of the American Chemical Society. | |
Explanation | The data from CAS Common Chemistry is provided under a CC-BY-NC 4.0 license, unless otherwise stated. | |
Record name | 2-Methyl-2-oxazoline | |
Source | ChemIDplus | |
URL | https://pubchem.ncbi.nlm.nih.gov/substance/?source=chemidplus&sourceid=0001120645 | |
Description | ChemIDplus is a free, web search system that provides access to the structure and nomenclature authority files used for the identification of chemical substances cited in National Library of Medicine (NLM) databases, including the TOXNET system. | |
Record name | 1120-64-5 | |
Source | DTP/NCI | |
URL | https://dtp.cancer.gov/dtpstandard/servlet/dwindex?searchtype=NSC&outputformat=html&searchlist=43141 | |
Description | The NCI Development Therapeutics Program (DTP) provides services and resources to the academic and private-sector research communities worldwide to facilitate the discovery and development of new cancer therapeutic agents. | |
Explanation | Unless otherwise indicated, all text within NCI products is free of copyright and may be reused without our permission. Credit the National Cancer Institute as the source. | |
Record name | 2-Methyl-2-oxazoline | |
Source | EPA DSSTox | |
URL | https://comptox.epa.gov/dashboard/DTXSID40870845 | |
Description | DSSTox provides a high quality public chemistry resource for supporting improved predictive toxicology. | |
Record name | 2-methyl-2-oxazoline | |
Source | European Chemicals Agency (ECHA) | |
URL | https://echa.europa.eu/substance-information/-/substanceinfo/100.013.016 | |
Description | The European Chemicals Agency (ECHA) is an agency of the European Union which is the driving force among regulatory authorities in implementing the EU's groundbreaking chemicals legislation for the benefit of human health and the environment as well as for innovation and competitiveness. | |
Explanation | Use of the information, documents and data from the ECHA website is subject to the terms and conditions of this Legal Notice, and subject to other binding limitations provided for under applicable law, the information, documents and data made available on the ECHA website may be reproduced, distributed and/or used, totally or in part, for non-commercial purposes provided that ECHA is acknowledged as the source: "Source: European Chemicals Agency, http://echa.europa.eu/". Such acknowledgement must be included in each copy of the material. ECHA permits and encourages organisations and individuals to create links to the ECHA website under the following cumulative conditions: Links can only be made to webpages that provide a link to the Legal Notice page. | |
Synthesis routes and methods
Procedure details
Retrosynthesis Analysis
AI-Powered Synthesis Planning: Our tool employs the Template_relevance Pistachio, Template_relevance Bkms_metabolic, Template_relevance Pistachio_ringbreaker, Template_relevance Reaxys, Template_relevance Reaxys_biocatalysis model, leveraging a vast database of chemical reactions to predict feasible synthetic routes.
One-Step Synthesis Focus: Specifically designed for one-step synthesis, it provides concise and direct routes for your target compounds, streamlining the synthesis process.
Accurate Predictions: Utilizing the extensive PISTACHIO, BKMS_METABOLIC, PISTACHIO_RINGBREAKER, REAXYS, REAXYS_BIOCATALYSIS database, our tool offers high-accuracy predictions, reflecting the latest in chemical research and data.
Strategy Settings
Precursor scoring | Relevance Heuristic |
---|---|
Min. plausibility | 0.01 |
Model | Template_relevance |
Template Set | Pistachio/Bkms_metabolic/Pistachio_ringbreaker/Reaxys/Reaxys_biocatalysis |
Top-N result to add to graph | 6 |
Feasible Synthetic Routes
Descargo de responsabilidad e información sobre productos de investigación in vitro
Tenga en cuenta que todos los artículos e información de productos presentados en BenchChem están destinados únicamente con fines informativos. Los productos disponibles para la compra en BenchChem están diseñados específicamente para estudios in vitro, que se realizan fuera de organismos vivos. Los estudios in vitro, derivados del término latino "in vidrio", involucran experimentos realizados en entornos de laboratorio controlados utilizando células o tejidos. Es importante tener en cuenta que estos productos no se clasifican como medicamentos y no han recibido la aprobación de la FDA para la prevención, tratamiento o cura de ninguna condición médica, dolencia o enfermedad. Debemos enfatizar que cualquier forma de introducción corporal de estos productos en humanos o animales está estrictamente prohibida por ley. Es esencial adherirse a estas pautas para garantizar el cumplimiento de los estándares legales y éticos en la investigación y experimentación.