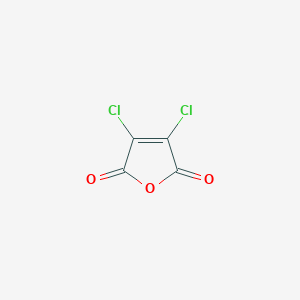
Dichloromaleic anhydride
Descripción general
Descripción
Métodos De Preparación
Rutas Sintéticas y Condiciones de Reacción: El alcohol elaídico se puede sintetizar mediante la hidrogenación selectiva del ácido oleico utilizando un catalizador de rutenio-estaño-alúmina . Las condiciones de reacción óptimas incluyen una temperatura de 250°C y una presión de 5.6 MPa, lo que resulta en altos rendimientos de 9-octadecen-1-ol (alcohol oleílico y elaídico) . Los métodos de preparación del catalizador, incluida la sol-gel, la impregnación y la coprecipitación, afectan significativamente la actividad y la selectividad del catalizador .
Métodos de Producción Industrial: En entornos industriales, el alcohol elaídico se produce mediante la hidrogenación del ácido elaídico . Este proceso implica el uso de gas hidrógeno y un catalizador metálico, típicamente paladio o níquel, en condiciones controladas de temperatura y presión .
Análisis De Reacciones Químicas
Tipos de Reacciones: El alcohol elaídico experimenta diversas reacciones químicas, incluyendo:
Reactivos y Condiciones Comunes:
Oxidación: Permanganato de potasio, trióxido de cromo y otros agentes oxidantes fuertes.
Reducción: Hidruro de litio y aluminio, borohidruro de sodio.
Sustitución: Diversos agentes halogenantes y ácidos.
Productos Principales:
Oxidación: Ácido elaídico.
Reducción: Alcohol esteárico.
Sustitución: Derivados halogenados y otros compuestos sustituidos.
Aplicaciones Científicas De Investigación
Chemical Properties and Structure
Dichloromaleic anhydride has the molecular formula and a molecular weight of approximately 166.94 g/mol. It exists as a solid at room temperature, with a melting point ranging from 118°C to 122°C . The compound is characterized by its reactive anhydride functional group, which facilitates various chemical reactions.
Organic Synthesis
DCMA is utilized as an intermediate in the synthesis of various organic compounds. Its reactivity allows it to participate in addition reactions with nucleophiles, making it valuable in creating complex molecules.
Case Study: Synthesis of Pyridine N-Oxides
Recent research has demonstrated the effectiveness of DCMA as a catalyst for the N-oxidation of pyridine derivatives using hydrogen peroxide. The study showed that DCMA can achieve high conversion rates for electron-rich substrates, highlighting its utility in organic synthesis .
Table 1: Catalytic Activity of DCMA in Pyridine N-Oxidation
Substrate | Conversion Rate (%) |
---|---|
Quinoline | 70 |
2-Picolinic Acid | High |
Electron-deficient | Low |
Polymer Chemistry
DCMA serves as a monomer in the production of various polymers. Its ability to undergo polymerization reactions allows it to contribute to the formation of copolymers with enhanced properties.
Application: Production of Copolymers
In polymer studies, DCMA has been used to synthesize copolymers that exhibit improved thermal stability and mechanical properties compared to traditional polymers. The incorporation of dichloromaleic units into polymer chains can significantly alter their physical properties, making them suitable for specialized applications .
Table 2: Properties of DCMA-based Polymers
Property | Value |
---|---|
Thermal Stability | High |
Mechanical Strength | Enhanced |
Solubility | Variable |
Catalytic Applications
DCMA is explored as a catalyst in various oxidation reactions, providing an environmentally friendly alternative to traditional oxidants.
Case Study: Oxidation Reactions
A study highlighted the catalytic performance of DCMA in oxidation reactions involving olefins and sulfides. The results indicated that DCMA could effectively catalyze these reactions while minimizing by-product formation, aligning with green chemistry principles .
Table 3: Oxidation Reactions Catalyzed by DCMA
Reaction Type | Substrate Type | Conversion Efficiency (%) |
---|---|---|
Olefin Epoxidation | Electron-rich | High |
Sulfide Oxidation | Various | Moderate |
Baeyer-Villiger Oxidation | Ketones | High |
Mecanismo De Acción
El alcohol elaídico ejerce sus efectos a través de varios mecanismos moleculares. Actúa como un agente antiviral al interrumpir las membranas lipídicas de los virus, inhibiendo así su capacidad para infectar las células huésped . El compuesto se dirige a la envoltura viral, lo que lleva a la inactivación del virus . Además, el alcohol elaídico puede interactuar con las membranas celulares, afectando la fluidez y la permeabilidad de la membrana .
Comparación Con Compuestos Similares
El alcohol elaídico es similar a otros alcoholes grasos monoinsaturados, como el alcohol oleílico (cis-9-octadecenol) y el alcohol palmitoleílico . es único debido a la presencia de un doble enlace trans, que le confiere diferentes propiedades químicas y físicas en comparación con sus contrapartes cis .
Compuestos Similares:
- Alcohol oleílico (cis-9-octadecenol) : Un alcohol graso monoinsaturado con un doble enlace cis.
- Alcohol palmitoleílico : Un alcohol graso monoinsaturado con un doble enlace cis en la séptima posición del carbono.
- Alcohol esteárico : Un alcohol graso saturado sin dobles enlaces.
La configuración trans del alcohol elaídico lo hace más rígido y menos flexible en comparación con los isómeros cis, lo que afecta su reactividad e interacciones con otras moléculas .
Actividad Biológica
Dichloromaleic anhydride (DCMA) is a chemical compound with the formula CClO. It is recognized for its diverse biological activities, particularly in antimicrobial and enzyme inhibition properties. This article explores the biological activity of DCMA, highlighting its mechanisms, applications, and relevant research findings.
DCMA is a derivative of maleic anhydride, characterized by the presence of two chlorine atoms. Its structure allows it to participate in various chemical reactions, making it a useful compound in organic synthesis and biological applications.
Antimicrobial Activity
Several studies have investigated the antimicrobial properties of DCMA. Research indicates that compounds containing maleic anhydride moieties, including DCMA, exhibit significant antibacterial and antifungal activities.
Case Study: Antimicrobial Efficacy
A study evaluated the antimicrobial activity of various cyclic imides derived from maleic anhydride derivatives against Gram-positive and Gram-negative bacteria. DCMA was included in this evaluation, demonstrating notable activity against Escherichia coli and Staphylococcus aureus. The minimum inhibitory concentrations (MICs) were determined, showcasing effective inhibition at low concentrations.
Compound | MIC (μg/mL) against E. coli | MIC (μg/mL) against S. aureus |
---|---|---|
This compound | 16 | 32 |
Phthalimide | 8 | 16 |
The results suggest that DCMA can inhibit bacterial growth effectively, supporting its potential use as an antimicrobial agent in various applications .
Enzyme Inhibition
DCMA has also been studied for its ability to inhibit specific enzymes. For instance, research has shown that it can inhibit protein phosphatases, which are crucial for various cellular processes. This inhibition can lead to altered cellular signaling pathways and may have implications in cancer research.
The mechanism by which DCMA exerts its effects involves the modification of enzyme active sites through covalent bonding. This irreversible binding alters enzyme functionality and can lead to significant biological effects.
Structure-Activity Relationship (SAR)
Understanding the structure-activity relationship of DCMA is vital for optimizing its biological activity. Modifications to the chlorine substituents or the anhydride structure can enhance or reduce its efficacy against specific pathogens or enzymes.
Research Findings on SAR
- Increased Activity : Substituting chlorine with other halogens has been shown to enhance antimicrobial properties.
- Decreased Toxicity : Altering the anhydride structure can reduce cytotoxicity while maintaining antimicrobial efficacy .
Applications in Biosensing
DCMA has been utilized in developing biosensors due to its ability to immobilize enzymes effectively. For example, horseradish peroxidase immobilized on surfaces treated with DCMA showed enhanced stability and activity for detecting catechol and resorcinol in aqueous solutions .
Propiedades
IUPAC Name |
3,4-dichlorofuran-2,5-dione | |
---|---|---|
Source | PubChem | |
URL | https://pubchem.ncbi.nlm.nih.gov | |
Description | Data deposited in or computed by PubChem | |
InChI |
InChI=1S/C4Cl2O3/c5-1-2(6)4(8)9-3(1)7 | |
Source | PubChem | |
URL | https://pubchem.ncbi.nlm.nih.gov | |
Description | Data deposited in or computed by PubChem | |
InChI Key |
AGULWIQIYWWFBJ-UHFFFAOYSA-N | |
Source | PubChem | |
URL | https://pubchem.ncbi.nlm.nih.gov | |
Description | Data deposited in or computed by PubChem | |
Canonical SMILES |
C1(=C(C(=O)OC1=O)Cl)Cl | |
Source | PubChem | |
URL | https://pubchem.ncbi.nlm.nih.gov | |
Description | Data deposited in or computed by PubChem | |
Molecular Formula |
C4Cl2O3 | |
Source | PubChem | |
URL | https://pubchem.ncbi.nlm.nih.gov | |
Description | Data deposited in or computed by PubChem | |
DSSTOX Substance ID |
DTXSID1061526 | |
Record name | Dichloromaleic anhydride | |
Source | EPA DSSTox | |
URL | https://comptox.epa.gov/dashboard/DTXSID1061526 | |
Description | DSSTox provides a high quality public chemistry resource for supporting improved predictive toxicology. | |
Molecular Weight |
166.94 g/mol | |
Source | PubChem | |
URL | https://pubchem.ncbi.nlm.nih.gov | |
Description | Data deposited in or computed by PubChem | |
Physical Description |
Beige crystalline solid; [Sigma-Aldrich MSDS] | |
Record name | Dichloromaleic anhydride | |
Source | Haz-Map, Information on Hazardous Chemicals and Occupational Diseases | |
URL | https://haz-map.com/Agents/20570 | |
Description | Haz-Map® is an occupational health database designed for health and safety professionals and for consumers seeking information about the adverse effects of workplace exposures to chemical and biological agents. | |
Explanation | Copyright (c) 2022 Haz-Map(R). All rights reserved. Unless otherwise indicated, all materials from Haz-Map are copyrighted by Haz-Map(R). No part of these materials, either text or image may be used for any purpose other than for personal use. Therefore, reproduction, modification, storage in a retrieval system or retransmission, in any form or by any means, electronic, mechanical or otherwise, for reasons other than personal use, is strictly prohibited without prior written permission. | |
CAS No. |
1122-17-4 | |
Record name | Dichloromaleic anhydride | |
Source | CAS Common Chemistry | |
URL | https://commonchemistry.cas.org/detail?cas_rn=1122-17-4 | |
Description | CAS Common Chemistry is an open community resource for accessing chemical information. Nearly 500,000 chemical substances from CAS REGISTRY cover areas of community interest, including common and frequently regulated chemicals, and those relevant to high school and undergraduate chemistry classes. This chemical information, curated by our expert scientists, is provided in alignment with our mission as a division of the American Chemical Society. | |
Explanation | The data from CAS Common Chemistry is provided under a CC-BY-NC 4.0 license, unless otherwise stated. | |
Record name | Dichloromaleic anhydride | |
Source | ChemIDplus | |
URL | https://pubchem.ncbi.nlm.nih.gov/substance/?source=chemidplus&sourceid=0001122174 | |
Description | ChemIDplus is a free, web search system that provides access to the structure and nomenclature authority files used for the identification of chemical substances cited in National Library of Medicine (NLM) databases, including the TOXNET system. | |
Record name | Dichloromaleic anhydride | |
Source | DTP/NCI | |
URL | https://dtp.cancer.gov/dtpstandard/servlet/dwindex?searchtype=NSC&outputformat=html&searchlist=60524 | |
Description | The NCI Development Therapeutics Program (DTP) provides services and resources to the academic and private-sector research communities worldwide to facilitate the discovery and development of new cancer therapeutic agents. | |
Explanation | Unless otherwise indicated, all text within NCI products is free of copyright and may be reused without our permission. Credit the National Cancer Institute as the source. | |
Record name | 2,5-Furandione, 3,4-dichloro- | |
Source | EPA Chemicals under the TSCA | |
URL | https://www.epa.gov/chemicals-under-tsca | |
Description | EPA Chemicals under the Toxic Substances Control Act (TSCA) collection contains information on chemicals and their regulations under TSCA, including non-confidential content from the TSCA Chemical Substance Inventory and Chemical Data Reporting. | |
Record name | Dichloromaleic anhydride | |
Source | EPA DSSTox | |
URL | https://comptox.epa.gov/dashboard/DTXSID1061526 | |
Description | DSSTox provides a high quality public chemistry resource for supporting improved predictive toxicology. | |
Record name | Dichloromaleic anhydride | |
Source | European Chemicals Agency (ECHA) | |
URL | https://echa.europa.eu/substance-information/-/substanceinfo/100.013.040 | |
Description | The European Chemicals Agency (ECHA) is an agency of the European Union which is the driving force among regulatory authorities in implementing the EU's groundbreaking chemicals legislation for the benefit of human health and the environment as well as for innovation and competitiveness. | |
Explanation | Use of the information, documents and data from the ECHA website is subject to the terms and conditions of this Legal Notice, and subject to other binding limitations provided for under applicable law, the information, documents and data made available on the ECHA website may be reproduced, distributed and/or used, totally or in part, for non-commercial purposes provided that ECHA is acknowledged as the source: "Source: European Chemicals Agency, http://echa.europa.eu/". Such acknowledgement must be included in each copy of the material. ECHA permits and encourages organisations and individuals to create links to the ECHA website under the following cumulative conditions: Links can only be made to webpages that provide a link to the Legal Notice page. | |
Record name | DICHLOROMALEIC ANHYDRIDE | |
Source | FDA Global Substance Registration System (GSRS) | |
URL | https://gsrs.ncats.nih.gov/ginas/app/beta/substances/56CE3A79HB | |
Description | The FDA Global Substance Registration System (GSRS) enables the efficient and accurate exchange of information on what substances are in regulated products. Instead of relying on names, which vary across regulatory domains, countries, and regions, the GSRS knowledge base makes it possible for substances to be defined by standardized, scientific descriptions. | |
Explanation | Unless otherwise noted, the contents of the FDA website (www.fda.gov), both text and graphics, are not copyrighted. They are in the public domain and may be republished, reprinted and otherwise used freely by anyone without the need to obtain permission from FDA. Credit to the U.S. Food and Drug Administration as the source is appreciated but not required. | |
Q1: What is the molecular formula and weight of dichloromaleic anhydride?
A1: this compound has the molecular formula C4Cl2O3 and a molecular weight of 166.94 g/mol. []
Q2: What spectroscopic techniques are useful for characterizing this compound?
A2: Researchers commonly employ infrared (IR) spectroscopy, nuclear magnetic resonance (NMR) spectroscopy, and mass spectrometry (MS) to characterize DCMA. [, , ] Additionally, UV-Vis spectroscopy is used to study its charge-transfer complexes and electronic transitions. [, ]
Q3: How does the presence of chlorine atoms in this compound affect its reactivity compared to maleic anhydride?
A3: The two chlorine atoms in DCMA increase its electron deficiency, making it a more potent dienophile in Diels-Alder reactions compared to maleic anhydride. [, , ] This enhanced reactivity makes DCMA a valuable building block for synthesizing complex molecules.
Q4: What type of reactions is this compound commonly used in?
A4: DCMA is frequently employed as a dienophile in Diels-Alder reactions, particularly in the synthesis of lactones and hydroisoindoles. [, ] It is also utilized in photochemical additions with benzene and polystyrene. []
Q5: Can you elaborate on the role of this compound in the synthesis of lactones?
A5: Research demonstrates that reacting DCMA with (E)-penta-2,4-dien-1-ol yields a triene intermediate, which upon heating undergoes intramolecular Diels-Alder cyclization to form a bicyclic acid. Subsequent dechlorodecarboxylation of this acid generates an unsaturated bicyclic lactone. []
Q6: How does the photochemical behavior of this compound differ from its thermal behavior?
A7: While photolysis of DCMA mainly leads to cycloaddition products, thermolysis predominantly generates dichloropropadienone, carbon monoxide (CO), and carbon dioxide (CO2). []
Q7: this compound has been investigated for its fungicidal properties. Can you elaborate on this?
A8: Researchers have synthesized chlorinated 1-arylamino-1H-pyrrole-2,5-diones, including those with 2′,4′-dichlorophenyl and 2′,4′,6′-trichlorophenyl substituents, using DCMA as a starting material. These compounds exhibited fungicidal activity in both in vitro and in vivo assays. []
Q8: What computational chemistry methods have been used to study this compound?
A10: Density functional theory (DFT) calculations, particularly at the B3LYP/6-31G(d,p) level, have been employed to explore the optimized molecular geometry of DCMA derivatives and to gain insights into reaction mechanisms. [] Additionally, LCAO-MO (linear combination of atomic orbitals-molecular orbital) approximations have been used to correlate experimental observations with theoretical parameters. []
Q9: Is this compound used in polymer synthesis?
A11: Yes, DCMA serves as a building block for various polymers. For instance, researchers have synthesized bisdichloromaleimides with different aromatic structures using DCMA as a starting material. These compounds were then polymerized by nucleophilic displacement of chlorine with 9,9-bis(p-aminophenyl)fluorene, yielding polymers with high anaerobic char yields. []
Q10: How does the structure of the diamine used in the synthesis of bisdichloromaleimides affect the thermal stability of the resulting polymers?
A12: Studies have shown that the thermal and thermooxidative stability of the cured resins derived from bisdichloromaleimides is influenced by the diamine employed in the imidization process. The order of relative thermal stability, from highest to lowest, is as follows: p-phenylenediamine > 4-aminophenyl ether > 4,4′-diaminodiphenylmethane > 4,4′-diaminodiphenylsulfone > hexamethylenediamine. []
Descargo de responsabilidad e información sobre productos de investigación in vitro
Tenga en cuenta que todos los artículos e información de productos presentados en BenchChem están destinados únicamente con fines informativos. Los productos disponibles para la compra en BenchChem están diseñados específicamente para estudios in vitro, que se realizan fuera de organismos vivos. Los estudios in vitro, derivados del término latino "in vidrio", involucran experimentos realizados en entornos de laboratorio controlados utilizando células o tejidos. Es importante tener en cuenta que estos productos no se clasifican como medicamentos y no han recibido la aprobación de la FDA para la prevención, tratamiento o cura de ninguna condición médica, dolencia o enfermedad. Debemos enfatizar que cualquier forma de introducción corporal de estos productos en humanos o animales está estrictamente prohibida por ley. Es esencial adherirse a estas pautas para garantizar el cumplimiento de los estándares legales y éticos en la investigación y experimentación.