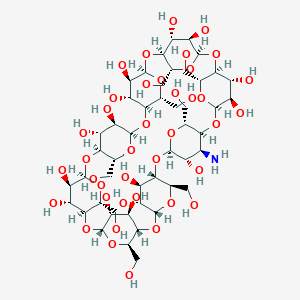
3A-Amino-3A-deoxy-(2AS,3AS)-gamma-cyclodextrin
Descripción general
Descripción
3A-Amino-3A-deoxy-(2AS,3AS)-gamma-cyclodextrin is a modified cyclodextrin compound where one of the glucose units in the cyclodextrin ring is replaced by an altro-pyranose unit with an amino group at the 3A position. Cyclodextrins are cyclic oligosaccharides composed of glucose units linked by alpha-1,4-glycosidic bonds. They are known for their ability to form inclusion complexes with various guest molecules due to their hydrophobic cavity and hydrophilic exterior .
Métodos De Preparación
Synthetic Routes and Reaction Conditions
The synthesis of 3A-Amino-3A-deoxy-(2AS,3AS)-gamma-cyclodextrin involves the selective modification of gamma-cyclodextrin. The process typically starts with the protection of hydroxyl groups followed by the introduction of the amino group at the 3A position. The reaction conditions often involve the use of protecting groups, such as acetyl or benzyl groups, to prevent unwanted reactions at other positions. The amino group can be introduced through nucleophilic substitution reactions using reagents like ammonia or amines .
Industrial Production Methods
Industrial production of this compound may involve large-scale synthesis using optimized reaction conditions to ensure high yield and purity. The process includes the use of automated reactors and purification techniques such as chromatography and crystallization to isolate the desired product .
Análisis De Reacciones Químicas
Types of Reactions
3A-Amino-3A-deoxy-(2AS,3AS)-gamma-cyclodextrin undergoes various chemical reactions, including:
Oxidation: The amino group can be oxidized to form nitro or nitroso derivatives.
Reduction: The compound can be reduced to form primary amines.
Substitution: The amino group can participate in nucleophilic substitution reactions to form derivatives with different functional groups.
Common Reagents and Conditions
Oxidation: Reagents such as hydrogen peroxide or nitric acid can be used under controlled conditions.
Reduction: Reducing agents like lithium aluminum hydride or sodium borohydride are commonly used.
Substitution: Nucleophiles such as alkyl halides or acyl chlorides can be used in the presence of a base.
Major Products Formed
The major products formed from these reactions include various substituted cyclodextrin derivatives with altered chemical and physical properties, which can be tailored for specific applications .
Aplicaciones Científicas De Investigación
Applications in Drug Delivery Systems
One of the most significant applications of 3A-Amino-3A-deoxy-(2AS,3AS)-gamma-cyclodextrin is in drug delivery systems . Its properties enhance the solubility and stability of poorly soluble drugs, leading to improved bioavailability.
Case Study: Encapsulation of Hydrophobic Drugs
Research has demonstrated that this cyclodextrin derivative can encapsulate hydrophobic drugs effectively. For instance, studies have shown that it can significantly increase the solubility of compounds like curcumin and paclitaxel, which are known for their poor aqueous solubility. By forming inclusion complexes, the compound not only enhances solubility but also protects the active ingredients from degradation .
Food Industry Applications
In the food industry, this compound is used as a food additive to improve flavor stability and control the release of flavors in food products. This application enhances consumer taste experiences and prolongs shelf life.
Table: Food Applications of Cyclodextrins
Application Type | Description |
---|---|
Flavor Stabilization | Enhances the stability of volatile flavor compounds. |
Controlled Release | Regulates the release of flavors during consumption. |
Texture Improvement | Modifies texture by encapsulating fat-soluble ingredients. |
Cosmetic Formulations
In cosmetics, this compound serves as a stabilizer and carrier for active ingredients. Its ability to improve skin absorption makes it crucial for enhancing the effectiveness of skincare products.
Case Study: Enhanced Skin Absorption
Research indicates that formulations containing this compound show improved penetration of active ingredients such as vitamins and antioxidants into the skin, thereby enhancing their efficacy .
Environmental Remediation
The compound has potential applications in environmental science for pollutant removal from water sources. It can form complexes with various contaminants, aiding in their extraction and degradation.
Example: Heavy Metal Removal
Studies have shown that this cyclodextrin derivative can effectively bind heavy metals such as lead and cadmium from contaminated water sources, facilitating their removal through adsorption processes .
Research and Development
In academic and industrial research settings, this compound is utilized as a versatile tool for studying molecular interactions and developing new materials.
Applications in Nanotechnology
It plays a significant role in nanotechnology by serving as a host molecule in supramolecular chemistry for the formation of inclusion complexes with guest molecules .
Mecanismo De Acción
The mechanism of action of 3A-Amino-3A-deoxy-(2AS,3AS)-gamma-cyclodextrin involves the formation of inclusion complexes with guest molecules. The hydrophobic cavity of the cyclodextrin allows it to encapsulate hydrophobic molecules, while the hydrophilic exterior interacts with the surrounding environment. This property is exploited in various applications, such as drug delivery, where the compound can enhance the solubility and stability of hydrophobic drugs .
Comparación Con Compuestos Similares
Similar Compounds
- 3A-Amino-3A-deoxy-(2AS,3AS)-alpha-cyclodextrin
- 3A-Amino-3A-deoxy-(2AS,3AS)-beta-cyclodextrin
- 6A-Amino-6A-deoxy-cyclodextrin
Uniqueness
3A-Amino-3A-deoxy-(2AS,3AS)-gamma-cyclodextrin is unique due to its specific structural modification, which imparts distinct chemical and physical properties. Compared to its alpha and beta counterparts, the gamma-cyclodextrin variant has a larger cavity size, allowing it to encapsulate larger guest molecules. This makes it particularly useful in applications requiring the inclusion of larger or more complex molecules .
Actividad Biológica
3A-Amino-3A-deoxy-(2AS,3AS)-gamma-cyclodextrin (3A-Amino-γ-CyD) is a modified cyclodextrin that has garnered attention due to its unique structural properties and biological activities. Cyclodextrins are cyclic oligosaccharides composed of glucose units, known for their ability to form inclusion complexes with various guest molecules. This article delves into the biological activity of 3A-Amino-γ-CyD, exploring its mechanisms of action, biochemical properties, and applications in various fields.
Structural Characteristics
The structural modification of 3A-Amino-γ-CyD involves the substitution of one glucose unit in the gamma-cyclodextrin ring with an altro-pyranose unit featuring an amino group at the 3A position. This modification enhances its solubility and interaction capabilities, making it an effective host for guest molecules. The compound is characterized by its ability to encapsulate hydrophobic compounds within its hydrophobic cavity while maintaining a hydrophilic exterior.
Host-Guest Interaction
The primary mechanism of action for 3A-Amino-γ-CyD is through host-guest complex formation. The amino group facilitates interactions with various biomolecules, enhancing the solubility and stability of poorly soluble drugs. This property is particularly valuable in pharmaceutical applications, where improved bioavailability can significantly impact therapeutic efficacy.
Biochemical Pathways
In biochemical reactions, 3A-Amino-γ-CyD plays a significant role in forming inclusion complexes with guest molecules in both aqueous and solid states. The molecular interactions are primarily driven by hydrophobic effects and hydrogen bonding, allowing for the selective encapsulation of target compounds.
Pharmacokinetics
Cyclodextrins, including 3A-Amino-γ-CyD, are known to enhance drug solubility and stability. For instance, studies have shown that this compound can improve the solubility of hydrophobic drugs like ibuprofen through the formation of stable inclusion complexes.
Cellular Effects
Research indicates that cyclodextrins can influence cell function by interacting with cell membranes. The incorporation of amino groups in 3A-Amino-γ-CyD enhances its ability to modulate cellular processes, potentially affecting drug delivery systems and therapeutic outcomes .
Case Studies
- Drug Delivery Applications : A study demonstrated that 3A-Amino-γ-CyD effectively enhanced the solubility and bioavailability of ibuprofen through complexation, showcasing its potential as a drug delivery agent.
- Chiral Recognition : Another investigation highlighted the use of 3A-Amino-γ-CyD as a chiral selector in electrokinetic chromatography for separating enantiomers, indicating its utility in analytical chemistry .
Research Findings
Recent studies have characterized the structural properties of 3A-Amino-γ-CyD using NMR spectroscopy, revealing detailed information about its hydrogen-bonding network and adaptability as a host molecule . The pKa values obtained from titration experiments suggest that the amino group can participate in protonation-deprotonation reactions under physiological conditions, further influencing its biological activity.
Pharmaceutical Industry
The ability to form inclusion complexes makes 3A-Amino-γ-CyD valuable in pharmaceutical formulations aimed at enhancing drug delivery systems. Its application extends to improving the solubility and stability of various therapeutic agents.
Material Science
In material science, this cyclodextrin derivative serves as a scaffold for hetero-functionalization, allowing for the development of novel materials with tailored properties .
Propiedades
IUPAC Name |
(1S,3R,5R,6S,8S,10R,11S,13R,15R,16S,18R,20R,21S,23R,25R,26S,28R,30R,31S,33R,35R,36S,38R,40R,41R,42R,43R,44R,45R,46R,47R,48R,49R,50R,51R,52R,53R,54R,55S,56S)-55-amino-5,10,15,20,25,30,35,40-octakis(hydroxymethyl)-2,4,7,9,12,14,17,19,22,24,27,29,32,34,37,39-hexadecaoxanonacyclo[36.2.2.23,6.28,11.213,16.218,21.223,26.228,31.233,36]hexapentacontane-41,42,43,44,45,46,47,48,49,50,51,52,53,54,56-pentadecol | |
---|---|---|
Source | PubChem | |
URL | https://pubchem.ncbi.nlm.nih.gov | |
Description | Data deposited in or computed by PubChem | |
InChI |
InChI=1S/C48H81NO39/c49-17-18(58)41-73-9(1-50)33(17)81-42-26(66)19(59)35(11(3-52)74-42)83-44-28(68)21(61)37(13(5-54)76-44)85-46-30(70)23(63)39(15(7-56)78-46)87-48-32(72)25(65)40(16(8-57)80-48)88-47-31(71)24(64)38(14(6-55)79-47)86-45-29(69)22(62)36(12(4-53)77-45)84-43-27(67)20(60)34(82-41)10(2-51)75-43/h9-48,50-72H,1-8,49H2/t9-,10-,11-,12-,13-,14-,15-,16-,17+,18+,19-,20-,21-,22-,23-,24-,25-,26-,27-,28-,29-,30-,31-,32-,33-,34-,35-,36-,37-,38-,39-,40-,41-,42-,43-,44-,45-,46-,47-,48-/m1/s1 | |
Source | PubChem | |
URL | https://pubchem.ncbi.nlm.nih.gov | |
Description | Data deposited in or computed by PubChem | |
InChI Key |
UCNMOVNZUVXILW-OJHPOGNJSA-N | |
Source | PubChem | |
URL | https://pubchem.ncbi.nlm.nih.gov | |
Description | Data deposited in or computed by PubChem | |
Canonical SMILES |
C(C1C2C(C(C(O1)OC3C(OC(C(C3O)O)OC4C(OC(C(C4O)O)OC5C(OC(C(C5O)O)OC6C(OC(C(C6O)O)OC7C(OC(C(C7O)O)OC8C(OC(C(C8O)O)OC9C(OC(O2)C(C9O)O)CO)CO)CO)CO)CO)CO)CO)O)N)O | |
Source | PubChem | |
URL | https://pubchem.ncbi.nlm.nih.gov | |
Description | Data deposited in or computed by PubChem | |
Isomeric SMILES |
C([C@@H]1[C@@H]2[C@H]([C@@H]([C@H](O1)O[C@@H]3[C@H](O[C@@H]([C@@H]([C@H]3O)O)O[C@@H]4[C@H](O[C@@H]([C@@H]([C@H]4O)O)O[C@@H]5[C@H](O[C@@H]([C@@H]([C@H]5O)O)O[C@@H]6[C@H](O[C@@H]([C@@H]([C@H]6O)O)O[C@@H]7[C@H](O[C@@H]([C@@H]([C@H]7O)O)O[C@@H]8[C@H](O[C@@H]([C@@H]([C@H]8O)O)O[C@@H]9[C@H](O[C@H](O2)[C@@H]([C@H]9O)O)CO)CO)CO)CO)CO)CO)CO)O)N)O | |
Source | PubChem | |
URL | https://pubchem.ncbi.nlm.nih.gov | |
Description | Data deposited in or computed by PubChem | |
Molecular Formula |
C48H81NO39 | |
Source | PubChem | |
URL | https://pubchem.ncbi.nlm.nih.gov | |
Description | Data deposited in or computed by PubChem | |
DSSTOX Substance ID |
DTXSID10468152 | |
Record name | 3A-Amino-3A-deoxy-(2AS,3AS)-gamma-cyclodextrin | |
Source | EPA DSSTox | |
URL | https://comptox.epa.gov/dashboard/DTXSID10468152 | |
Description | DSSTox provides a high quality public chemistry resource for supporting improved predictive toxicology. | |
Molecular Weight |
1296.1 g/mol | |
Source | PubChem | |
URL | https://pubchem.ncbi.nlm.nih.gov | |
Description | Data deposited in or computed by PubChem | |
CAS No. |
189307-64-0 | |
Record name | 3A-Amino-3A-deoxy-(2AS,3AS)-gamma-cyclodextrin | |
Source | EPA DSSTox | |
URL | https://comptox.epa.gov/dashboard/DTXSID10468152 | |
Description | DSSTox provides a high quality public chemistry resource for supporting improved predictive toxicology. | |
Descargo de responsabilidad e información sobre productos de investigación in vitro
Tenga en cuenta que todos los artículos e información de productos presentados en BenchChem están destinados únicamente con fines informativos. Los productos disponibles para la compra en BenchChem están diseñados específicamente para estudios in vitro, que se realizan fuera de organismos vivos. Los estudios in vitro, derivados del término latino "in vidrio", involucran experimentos realizados en entornos de laboratorio controlados utilizando células o tejidos. Es importante tener en cuenta que estos productos no se clasifican como medicamentos y no han recibido la aprobación de la FDA para la prevención, tratamiento o cura de ninguna condición médica, dolencia o enfermedad. Debemos enfatizar que cualquier forma de introducción corporal de estos productos en humanos o animales está estrictamente prohibida por ley. Es esencial adherirse a estas pautas para garantizar el cumplimiento de los estándares legales y éticos en la investigación y experimentación.