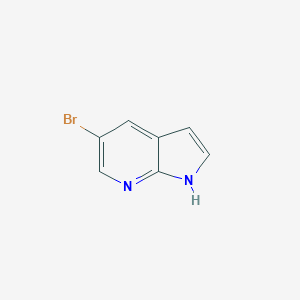
5-Bromo-7-azaindole
Descripción general
Descripción
5-Bromo-7-azaindole (C₇H₅BrN₂; CAS 183208-35-7) is a halogenated heterocyclic compound notable for its role as a key intermediate in pharmaceutical synthesis, particularly for the BRAF inhibitor Vemurafenib . Structurally, it features a bromine substituent at the 5-position of the 7-azaindole scaffold, a pyrrolopyridine derivative. Its synthesis typically involves catalytic hydrogenation of 7-azaindole followed by regioselective bromination and oxidative dehydrogenation, achieving yields >74% with high purity (≥99%) . This compound’s reactivity at the C5 position enables diverse functionalization, making it pivotal in medicinal chemistry and materials science.
Métodos De Preparación
Traditional Synthesis from 2-Aminopyridine Derivatives
Bromination-Cyclization Route
The most cited method involves brominating 2-aminopyridine followed by cyclization. As detailed in CN108084076A , this two-step process begins with bromination using phosphorus oxychloride (POCl₃) or N-bromosuccinimide (NBS) in dimethyl sulfoxide (DMSO). The intermediate 2-amino-5-bromopyridine undergoes cyclization with acetaldehyde under acidic conditions (concentrated H₂SO₄) at 70–90°C . A catalyst mixture of phosphorus oxychloride, copper acetate, and niobium pentachloride (4:3:1 molar ratio) enhances reaction efficiency, achieving 85–90% yield .
Key Challenges:
-
Bromine Handling : Excess bromine necessitates careful quenching to prevent side reactions .
-
Byproduct Formation : Over-bromination at the C-3 position occurs without precise stoichiometry, requiring chromatographic purification .
Hydrazine-Mediated Cyclization
An alternative approach from CN109232563A employs 2-hydrazino-5-bromopyridine as the starting material. Reaction with acetaldehyde in DMF/DMSO at 80–85°C produces this compound via Fischer indole synthesis . Sodium carbonate neutralizes liberated ammonia, while anhydrous sodium sulfate absorbs water to shift equilibrium .
Optimization Data:
Parameter | Optimal Value | Impact on Yield |
---|---|---|
Temperature | 85°C | Maximizes cyclization rate |
Acetaldehyde Ratio | 1:1.2 (substrate) | Minimizes dimerization |
Catalyst Loading | 19:15 (substrate) | Prevents over-oxidation |
This method achieves 75–80% yield but requires strict exclusion of moisture to avoid hydrolysis .
Palladium/Copper-Catalyzed Cyclization
Reaction Mechanism
A high-yielding protocol from Ambeed utilizes bis(triphenylphosphine)palladium(II) dichloride and copper(I) iodide to mediate cyclization of 2-amino-5-bromo-3-iodopyridine. The reaction proceeds via Sonogashira coupling with 1,1-dimethyl-2-propyn-1-ol, followed by base-induced ring closure (Scheme 1) :
3\text{)}2 \xrightarrow{\text{Pd/Cu}} \text{this compound}
Advantages:
-
Single-Pot Synthesis : Eliminates intermediate isolation steps .
-
Scalability : Demonstrated at 50 kg scale with 90% yield and 99.3% HPLC purity .
Limitations:
-
Catalyst Cost : Palladium complexes increase production expenses.
-
Iodine Byproducts : Require extraction with toluene/water mixtures .
Catalyst-Free Methylenation with Aqueous Formaldehyde
Green Chemistry Approach
RSC Publishing reports a solvent-free method using 50% aqueous formaldehyde under microwave irradiation (5 min, 150°C). This pathway avoids metal catalysts but faces selectivity issues:
\text{N-Methyl-7-azaindole} + \text{HCHO} \rightarrow \text{N-Hydroxy methyl-7-azaindole} \quad (\text{44% yield})
Key Observations:
-
N-Methylation Requirement : Free N–H groups lead to exclusive N-hydroxy methyl adducts, necessitating pre-methylated substrates .
-
Microwave vs. Conventional Heating : Sealed-tube heating at 95°C improves yield to 95% but extends reaction time to 4 hours .
Industrial-Scale Synthesis via Alkoxide-Mediated Cyclization
Potassium tert-Butoxide Protocol
Ambeed’s large-scale method dissolves 2-amino-5-bromo-3-ethynylpyridine in DMF with potassium tert-butoxide at 60–85°C. Key parameters include:
Stage | Conditions | Outcome |
---|---|---|
Cyclization | 80–85°C, 3 h | 90% conversion |
Workup | Ethyl acetate/hexane | 99.3% purity |
Process Challenges:
-
Exothermic Reaction : Requires ice-water quenching to control temperature .
-
Filtration Difficulties : Diatomaceous earth aids solid separation .
Comparative Analysis of Methods
Yield and Scalability
Method | Yield (%) | Scalability | Cost Drivers |
---|---|---|---|
Bromination-Cyclization | 75–85 | Moderate | Bromine, catalysts |
Hydrazine Cyclization | 70–80 | Low | Moisture sensitivity |
Pd/Cu Catalyzed | 90 | High | Palladium complexes |
tert-Butoxide | 90 | Industrial | Temperature control |
Análisis De Reacciones Químicas
Types of Reactions:
Substitution Reactions: 5-Bromo-1H-pyrrolo[2,3-b]pyridine undergoes nucleophilic substitution reactions where the bromine atom is replaced by other nucleophiles such as amines, thiols, or alkoxides.
Oxidation and Reduction: The compound can be oxidized or reduced under specific conditions to form various derivatives.
Common Reagents and Conditions:
Nucleophilic Substitution: Reagents like sodium hydride (NaH), potassium carbonate (K2CO3), and solvents such as dimethylformamide (DMF) or tetrahydrofuran (THF) are commonly used.
Oxidation: Potassium permanganate (KMnO4) or chromium trioxide (CrO3) in acidic or basic conditions.
Major Products Formed:
Substitution Products: Various substituted pyrrolo[2,3-b]pyridine derivatives depending on the nucleophile used.
Oxidation Products: Oxidized derivatives such as pyrrolo[2,3-b]pyridine-2-carboxylic acid.
Aplicaciones Científicas De Investigación
Synthesis of 5-Bromo-7-Azaindole
The synthesis of this compound typically involves the bromination of 7-azaindole. A notable method includes using Raney nickel in an ethanol medium under hydrogen pressure, followed by bromination with bromine in the presence of p-toluenesulfonic acid . The overall reaction can be summarized as follows:
- Hydrogenation : 7-Azaindole is treated with Raney nickel and hydrogen.
- Bromination : The resulting product undergoes bromination to yield this compound.
This method is advantageous due to its high purity (>99%) and yield (>74%), making it suitable for large-scale production .
Biological Activities
This compound has garnered attention for its biological properties, particularly in cancer research and as an anti-parasitic agent.
Anticancer Activity
Research indicates that this compound exhibits significant anti-cancer properties. It has been evaluated against various cancer cell lines, demonstrating promising results in inhibiting cell proliferation. For example, studies involving the A2780 ovarian cancer cell line have shown that derivatives of this compound can effectively reduce cell viability .
Anti-Parasitic Activity
In addition to its anticancer properties, this compound has been identified as a growth inhibitor for Trypanosoma brucei, the causative agent of human African trypanosomiasis (HAT). High-throughput screening has revealed that certain derivatives exhibit potent anti-trypanosomal activity, although challenges remain concerning their ability to penetrate the blood-brain barrier .
Applications in Drug Development
The unique properties of this compound make it a valuable intermediate in the synthesis of various pharmaceuticals:
- Antitumor Drugs : Its derivatives are being explored as potential leads for new anticancer therapies.
- Neglected Tropical Diseases : Compounds derived from this compound are being investigated for their efficacy against neglected tropical diseases like HAT .
- Histone Deacetylase Inhibition : Some studies suggest that compounds based on this structure may act as inhibitors of histone deacetylases (HDACs), which are crucial targets in cancer therapy .
Case Study 1: Antitumor Efficacy
A study focused on the effects of this compound on A2780 and cisplatin-resistant A2780CisR cell lines showed that it significantly inhibited cell proliferation at concentrations below 10 µM. The results indicated a potential role in overcoming drug resistance in ovarian cancer treatments .
Case Study 2: Anti-Parasitic Activity
Another investigation into the anti-trypanosomal effects of 3,5-disubstituted derivatives of 7-azaindoles demonstrated that some compounds effectively inhibited the growth of T. brucei, with IC50 values indicating strong activity against the parasite. This highlights the compound's potential in developing new treatments for HAT .
Mecanismo De Acción
Molecular Targets and Pathways:
FGFR Inhibition: 5-Bromo-1H-pyrrolo[2,3-b]pyridine derivatives have shown potent inhibitory activity against FGFR1, FGFR2, and FGFR3.
Apoptosis Induction: The compound induces apoptosis in cancer cells by inhibiting FGFR signaling pathways, leading to reduced cell proliferation and increased cell death.
Comparación Con Compuestos Similares
Comparison with Similar Halogenated 7-Azaindole Derivatives
Structural and Functional Differences
The biological and chemical properties of halogenated 7-azaindoles depend on the position and type of halogen substituent. Below is a comparative analysis:
Key Observations:
- Positional Influence : The C5 bromine in this compound enhances its utility in cross-coupling reactions (e.g., Suzuki-Miyaura) for synthesizing diarylated derivatives with improved kinase inhibition (e.g., DYRK1A inhibitors) . In contrast, C3-substituted derivatives (e.g., 3-Iodo-7-azaindole) exhibit superior antitumor activity in platinum(II) complexes, likely due to optimized steric and electronic interactions with DNA .
- Synthetic Versatility : The bromine at C5 allows sequential functionalization, as seen in PROTACs targeting BRAFV600E, where this compound serves as a scaffold for linker attachment .
Mechanistic Insights from Platinum Complex Studies
Platinum(II) oxalato complexes with 7-azaindole derivatives highlight substituent-dependent bioactivity:
- This disparity underscores the importance of halogen position in dictating DNA-binding affinity and cellular uptake.
Actividad Biológica
5-Bromo-7-azaindole is a heterocyclic compound that has garnered attention in medicinal chemistry due to its diverse biological activities. This article explores its synthesis, biological properties, structure-activity relationships (SAR), and potential therapeutic applications, particularly in the context of neglected tropical diseases and cancer.
1. Synthesis of this compound
This compound can be synthesized through various methods, including palladium-catalyzed cross-coupling reactions. The synthetic route typically involves the bromination of 7-azaindole derivatives, which can then be further functionalized to enhance biological activity. Recent studies have reported the successful synthesis of 5-bromo derivatives using commercially available starting materials, highlighting the accessibility of this compound for further research and development .
2.1 Antiparasitic Activity
One of the most significant biological activities of this compound is its antiparasitic effect against Trypanosoma brucei, the causative agent of human African trypanosomiasis (HAT). In a high-throughput screening study, several derivatives, including those based on the 7-azaindole core, were identified as potent growth inhibitors of T. brucei. Notably, compounds with a pEC50 greater than 7.0 were reported, indicating strong inhibitory effects .
Table 1 summarizes key findings regarding the activity of various analogs against T. brucei:
Compound ID | pEC50 | HLM Cl int (μg/min/mg protein) | Aqueous Solubility (μM) |
---|---|---|---|
NEU-1207 | >7.0 | 300 | 6 |
NEU-1208 | >7.0 | 180 | 13 |
NEU-1209 | <6.5 | >300 | 17 |
These results indicate that structural modifications at the 3 and 5 positions are crucial for maintaining activity against T. brucei .
2.2 Antitumor Activity
In addition to its antiparasitic properties, this compound has shown promise in cancer research. Studies have evaluated its effectiveness against various cancer cell lines, including osteosarcoma (HOS) and breast adenocarcinoma (MCF7). For instance, platinum(II) oxalato complexes incorporating this compound demonstrated moderate antitumor effects with IC50 values ranging from approximately 18.3 μM to over 27.5 μM .
3. Structure-Activity Relationships (SAR)
The SAR studies conducted on this compound derivatives reveal that modifications at specific positions significantly influence their biological activity. For example:
- Methylation or tosylation at the indole-NH position generally resulted in a loss of activity against T. brucei.
- Substituents at the 3 and 5 positions were found to be interchangeable without substantial loss in potency .
Case Study: Antiparasitic Screening
A recent study screened a library of azaindole derivatives for their effectiveness against T. brucei. The study identified several compounds with significant growth inhibition capabilities, leading to further optimization efforts aimed at enhancing pharmacokinetic properties while ensuring efficacy .
Case Study: Antitumor Efficacy
In another investigation focusing on cancer treatments, researchers synthesized various platinum complexes with different azaindole derivatives, including this compound. These complexes were tested for their cytotoxicity against HOS and MCF7 cells, revealing that certain substitutions could improve solubility and bioavailability while retaining antitumor activity .
Q & A
Basic Research Questions
Q. What are the common synthetic routes for 5-bromo-7-azaindole, and how can researchers optimize yields?
The synthesis of this compound typically involves a three-step route starting from 2-amino-3-methylpyridine. Key steps include bromination, hydroxymethylation, and cyclization. For example, bromination of 7-azaindole with molecular bromine (Br₂) in acetic acid at 0–5°C achieves regioselective substitution at the 5-position . Yield optimization requires careful control of reaction temperature, stoichiometry, and purification via recrystallization (e.g., using ethanol/water mixtures) .
Q. How is this compound utilized in pharmaceutical research?
this compound serves as a critical intermediate in synthesizing kinase inhibitors such as vemurafenib (used in melanoma therapy) and ABT-199 (a BCL-2 inhibitor). Its bromine atom enables cross-coupling reactions (e.g., Suzuki or Buchwald-Hartwig) to introduce aryl or heteroaryl groups essential for drug activity .
Q. What analytical techniques are recommended for characterizing this compound and its derivatives?
Essential techniques include:
- Melting point analysis : 179–181°C (lit. range) to confirm purity .
- HPLC : Purity >96% with C18 columns and acetonitrile/water mobile phases .
- Spectroscopy : ¹H/¹³C NMR (DMSO-d₆) for structural confirmation; IR for functional group identification .
Q. What safety protocols should be followed when handling this compound?
The compound is a crystalline powder with moderate solubility in DMSO and oxygenated solvents. Use PPE (gloves, goggles) to avoid inhalation or skin contact. Store in airtight containers at 2–8°C to prevent degradation .
Q. What are the most studied derivatives of this compound in medicinal chemistry?
Examples include:
- Bis-7-azaindolyl methanones : Synthesized via microwave-assisted coupling with aldehydes .
- 5-Aryl-3,3′-bis-indolyl derivatives : Prepared through palladium-catalyzed cross-coupling reactions .
Advanced Research Questions
Q. How can regioselectivity challenges in 7-azaindole bromination be addressed?
Regioselective bromination at the 5-position is achieved using Br₂ in acetic acid under controlled conditions (0–5°C). For complex substrates (e.g., 4-chloro-3-nitro-7-azaindole), bromination at the 5-position is favored due to electron-withdrawing group effects, enabling >95% regioselectivity .
Q. How should researchers resolve contradictions in reported synthetic yields or spectral data?
- Replicate conditions : Ensure identical reagents, temperatures, and purification methods.
- Validate sources : Cross-check with primary literature (e.g., Chem. Rev., J. Med. Chem.) and avoid unreliable databases .
- Use control experiments : Compare intermediates (e.g., 7-azaindoline) to isolate discrepancies .
Q. What green chemistry strategies apply to this compound synthesis?
- Microwave-assisted synthesis : Reduces reaction time (30 min vs. hours) and solvent use (MeOH/H₂O mixtures) .
- Catalyst recycling : Palladium catalysts (e.g., Pd/C) can be recovered via filtration .
Q. How can crystallographic data (e.g., CCDC entries) enhance understanding of 7-azaindole derivatives?
Crystal structures (e.g., CCDC-2191474) reveal hydrogen-bonding networks and π-stacking interactions, which inform solubility and reactivity. For example, planar 7-azaindole cores favor stacking in kinase binding pockets .
Q. What strategies improve the bioactivity of this compound derivatives?
- Structure-activity relationship (SAR) studies : Introduce electron-withdrawing groups (e.g., nitro, chloro) at the 3- or 4-position to enhance kinase inhibition .
- Prodrug design : Mask polar groups (e.g., carboxylic acids) to improve bioavailability .
Q. How can researchers ensure reproducibility in multi-step syntheses involving this compound?
- Detailed documentation : Record reaction parameters (e.g., stirring speed, cooling rates) .
- Intermediate characterization : Validate each step via TLC, NMR, or mass spectrometry .
Q. What functionalization strategies enable diversification of this compound?
Propiedades
IUPAC Name |
5-bromo-1H-pyrrolo[2,3-b]pyridine | |
---|---|---|
Source | PubChem | |
URL | https://pubchem.ncbi.nlm.nih.gov | |
Description | Data deposited in or computed by PubChem | |
InChI |
InChI=1S/C7H5BrN2/c8-6-3-5-1-2-9-7(5)10-4-6/h1-4H,(H,9,10) | |
Source | PubChem | |
URL | https://pubchem.ncbi.nlm.nih.gov | |
Description | Data deposited in or computed by PubChem | |
InChI Key |
LPTVWZSQAIDCEB-UHFFFAOYSA-N | |
Source | PubChem | |
URL | https://pubchem.ncbi.nlm.nih.gov | |
Description | Data deposited in or computed by PubChem | |
Canonical SMILES |
C1=CNC2=NC=C(C=C21)Br | |
Source | PubChem | |
URL | https://pubchem.ncbi.nlm.nih.gov | |
Description | Data deposited in or computed by PubChem | |
Molecular Formula |
C7H5BrN2 | |
Source | PubChem | |
URL | https://pubchem.ncbi.nlm.nih.gov | |
Description | Data deposited in or computed by PubChem | |
DSSTOX Substance ID |
DTXSID70437889 | |
Record name | 5-Bromo-7-azaindole | |
Source | EPA DSSTox | |
URL | https://comptox.epa.gov/dashboard/DTXSID70437889 | |
Description | DSSTox provides a high quality public chemistry resource for supporting improved predictive toxicology. | |
Molecular Weight |
197.03 g/mol | |
Source | PubChem | |
URL | https://pubchem.ncbi.nlm.nih.gov | |
Description | Data deposited in or computed by PubChem | |
CAS No. |
183208-35-7 | |
Record name | 5-Bromo-1H-pyrrolo[2,3-b]pyridine | |
Source | CAS Common Chemistry | |
URL | https://commonchemistry.cas.org/detail?cas_rn=183208-35-7 | |
Description | CAS Common Chemistry is an open community resource for accessing chemical information. Nearly 500,000 chemical substances from CAS REGISTRY cover areas of community interest, including common and frequently regulated chemicals, and those relevant to high school and undergraduate chemistry classes. This chemical information, curated by our expert scientists, is provided in alignment with our mission as a division of the American Chemical Society. | |
Explanation | The data from CAS Common Chemistry is provided under a CC-BY-NC 4.0 license, unless otherwise stated. | |
Record name | 5-Bromo-1H-pyrrolo(2,3-b)pyridine | |
Source | ChemIDplus | |
URL | https://pubchem.ncbi.nlm.nih.gov/substance/?source=chemidplus&sourceid=0183208357 | |
Description | ChemIDplus is a free, web search system that provides access to the structure and nomenclature authority files used for the identification of chemical substances cited in National Library of Medicine (NLM) databases, including the TOXNET system. | |
Record name | 5-Bromo-7-azaindole | |
Source | EPA DSSTox | |
URL | https://comptox.epa.gov/dashboard/DTXSID70437889 | |
Description | DSSTox provides a high quality public chemistry resource for supporting improved predictive toxicology. | |
Record name | 5-bromo-1H-pyrrolo[2,3-b]pyridine | |
Source | European Chemicals Agency (ECHA) | |
URL | https://echa.europa.eu/substance-information/-/substanceinfo/100.157.445 | |
Description | The European Chemicals Agency (ECHA) is an agency of the European Union which is the driving force among regulatory authorities in implementing the EU's groundbreaking chemicals legislation for the benefit of human health and the environment as well as for innovation and competitiveness. | |
Explanation | Use of the information, documents and data from the ECHA website is subject to the terms and conditions of this Legal Notice, and subject to other binding limitations provided for under applicable law, the information, documents and data made available on the ECHA website may be reproduced, distributed and/or used, totally or in part, for non-commercial purposes provided that ECHA is acknowledged as the source: "Source: European Chemicals Agency, http://echa.europa.eu/". Such acknowledgement must be included in each copy of the material. ECHA permits and encourages organisations and individuals to create links to the ECHA website under the following cumulative conditions: Links can only be made to webpages that provide a link to the Legal Notice page. | |
Synthesis routes and methods I
Procedure details
Synthesis routes and methods II
Procedure details
Synthesis routes and methods III
Procedure details
Synthesis routes and methods IV
Procedure details
Retrosynthesis Analysis
AI-Powered Synthesis Planning: Our tool employs the Template_relevance Pistachio, Template_relevance Bkms_metabolic, Template_relevance Pistachio_ringbreaker, Template_relevance Reaxys, Template_relevance Reaxys_biocatalysis model, leveraging a vast database of chemical reactions to predict feasible synthetic routes.
One-Step Synthesis Focus: Specifically designed for one-step synthesis, it provides concise and direct routes for your target compounds, streamlining the synthesis process.
Accurate Predictions: Utilizing the extensive PISTACHIO, BKMS_METABOLIC, PISTACHIO_RINGBREAKER, REAXYS, REAXYS_BIOCATALYSIS database, our tool offers high-accuracy predictions, reflecting the latest in chemical research and data.
Strategy Settings
Precursor scoring | Relevance Heuristic |
---|---|
Min. plausibility | 0.01 |
Model | Template_relevance |
Template Set | Pistachio/Bkms_metabolic/Pistachio_ringbreaker/Reaxys/Reaxys_biocatalysis |
Top-N result to add to graph | 6 |
Feasible Synthetic Routes
Descargo de responsabilidad e información sobre productos de investigación in vitro
Tenga en cuenta que todos los artículos e información de productos presentados en BenchChem están destinados únicamente con fines informativos. Los productos disponibles para la compra en BenchChem están diseñados específicamente para estudios in vitro, que se realizan fuera de organismos vivos. Los estudios in vitro, derivados del término latino "in vidrio", involucran experimentos realizados en entornos de laboratorio controlados utilizando células o tejidos. Es importante tener en cuenta que estos productos no se clasifican como medicamentos y no han recibido la aprobación de la FDA para la prevención, tratamiento o cura de ninguna condición médica, dolencia o enfermedad. Debemos enfatizar que cualquier forma de introducción corporal de estos productos en humanos o animales está estrictamente prohibida por ley. Es esencial adherirse a estas pautas para garantizar el cumplimiento de los estándares legales y éticos en la investigación y experimentación.