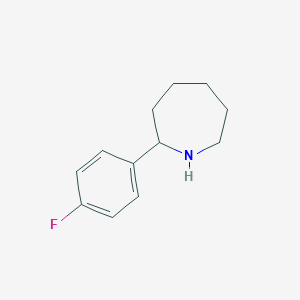
2-(4-Fluorofenil)azepan
- Haga clic en CONSULTA RÁPIDA para recibir una cotización de nuestro equipo de expertos.
- Con productos de calidad a un precio COMPETITIVO, puede centrarse más en su investigación.
Descripción general
Descripción
2-(4-Fluorophenyl)azepane is a chemical compound with the molecular formula C12H16FN. It is a seven-membered heterocyclic compound containing a fluorophenyl group attached to an azepane ring. This compound is primarily used for research and development purposes in various scientific fields.
Aplicaciones Científicas De Investigación
2-(4-Fluorophenyl)azepane has diverse applications in scientific research, including:
Chemistry: Used as a building block in the synthesis of more complex molecules and as a reagent in various organic reactions.
Biology: Employed in the study of biological pathways and interactions due to its structural similarity to biologically active compounds.
Medicine: Investigated for potential therapeutic applications, including as a precursor for drug development.
Industry: Utilized in the production of specialty chemicals and materials
Mecanismo De Acción
Target of Action
It is known that azepane derivatives, such as 2-(4-fluorophenyl)azepane, are often used in medicinal chemistry to explore the pharmacophore space due to their sp3-hybridization . This allows for a wide range of interactions with various biological targets.
Mode of Action
The stereochemistry of the molecule, contributed by the azepane ring, can lead to different biological profiles of drug candidates due to the different binding modes to enantioselective proteins .
Biochemical Pathways
Compounds with azepane rings are known to interact with various biological targets, potentially affecting multiple biochemical pathways .
Pharmacokinetics
It’s worth noting that the pharmacokinetics of a compound can be influenced by its chemical structure, and the azepane ring in 2-(4-fluorophenyl)azepane could potentially impact its bioavailability .
Result of Action
The presence of the azepane ring and the fluorophenyl group could potentially lead to a variety of biological effects, depending on the specific targets and pathways involved .
Métodos De Preparación
Synthetic Routes and Reaction Conditions
The synthesis of 2-(4-Fluorophenyl)azepane typically involves the reaction of 4-fluorobenzylamine with a suitable azepane precursor under controlled conditions. One common method includes the use of a base such as sodium hydride or potassium carbonate to facilitate the nucleophilic substitution reaction. The reaction is usually carried out in an organic solvent like dimethylformamide or tetrahydrofuran at elevated temperatures .
Industrial Production Methods
Industrial production of 2-(4-Fluorophenyl)azepane follows similar synthetic routes but on a larger scale. The process involves optimizing reaction conditions to ensure high yield and purity. Advanced techniques such as continuous flow reactors and automated synthesis systems are often employed to enhance efficiency and scalability .
Análisis De Reacciones Químicas
Types of Reactions
2-(4-Fluorophenyl)azepane undergoes various chemical reactions, including:
Oxidation: The compound can be oxidized using oxidizing agents like potassium permanganate or chromium trioxide to form corresponding ketones or carboxylic acids.
Reduction: Reduction reactions can be performed using reducing agents such as lithium aluminum hydride or sodium borohydride to yield amines or alcohols.
Substitution: Nucleophilic substitution reactions can occur at the fluorophenyl group, where the fluorine atom is replaced by other nucleophiles like amines or thiols.
Common Reagents and Conditions
Oxidation: Potassium permanganate in acidic or basic medium.
Reduction: Lithium aluminum hydride in anhydrous ether.
Substitution: Sodium hydride in dimethylformamide.
Major Products Formed
Oxidation: Formation of ketones or carboxylic acids.
Reduction: Formation of primary or secondary amines.
Substitution: Formation of substituted phenylazepanes.
Comparación Con Compuestos Similares
Similar Compounds
- 2-Phenylazepane
- 2-(4-Chlorophenyl)azepane
- 2-(4-Methylphenyl)azepane
Comparison
2-(4-Fluorophenyl)azepane is unique due to the presence of the fluorine atom, which imparts distinct electronic and steric properties. This fluorine substitution enhances the compound’s stability and reactivity compared to its analogs. Additionally, the fluorophenyl group increases its lipophilicity, improving its ability to cross biological membranes and interact with hydrophobic pockets in target proteins .
Actividad Biológica
2-(4-Fluorophenyl)azepane is a seven-membered heterocyclic compound characterized by the presence of a fluorophenyl group attached to an azepane ring. With the molecular formula C12H16FN, this compound has garnered interest in medicinal chemistry due to its potential biological activities and applications in drug development. This article explores the biological activity, mechanisms of action, and relevant research findings associated with 2-(4-Fluorophenyl)azepane.
Molecular Structure:
- Formula: C12H16FN
- Molecular Weight: 201.26 g/mol
- Functional Groups: Azepane ring, fluorophenyl group
Synthesis:
The synthesis of 2-(4-Fluorophenyl)azepane typically involves the reaction of 4-fluorobenzylamine with an azepane precursor. Common methods include:
- Nucleophilic substitution reactions using bases like sodium hydride or potassium carbonate.
- Solvents such as dimethylformamide (DMF) or tetrahydrofuran (THF) at elevated temperatures.
The biological activity of 2-(4-Fluorophenyl)azepane is largely influenced by its structural characteristics:
- Binding Affinity: The stereochemistry provided by the azepane ring allows for diverse binding modes to enantioselective proteins, potentially leading to varied pharmacological profiles.
- Biochemical Pathways: It interacts with multiple biological targets, influencing various pathways, including neurotransmitter systems and cellular signaling mechanisms.
Pharmacological Effects
Research indicates that compounds similar to 2-(4-Fluorophenyl)azepane exhibit a range of pharmacological effects:
- Antidepressant Activity: Studies have suggested that azepane derivatives can modulate serotonin and norepinephrine levels, contributing to their antidepressant effects .
- Cytotoxicity: In vitro assays have shown that related compounds can exhibit cytotoxic effects against various cancer cell lines, indicating potential anti-cancer properties .
Study on Antidepressant Properties
A study published in Organic & Biomolecular Chemistry evaluated the antidepressant-like effects of azepane derivatives, including 2-(4-Fluorophenyl)azepane. The results demonstrated significant improvements in behavioral tests indicative of antidepressant activity in animal models .
Cytotoxicity Assessment
Research conducted on a library of pharmacologically active compounds assessed the cytotoxic effects of 2-(4-Fluorophenyl)azepane on murine fibroblasts using the MTT assay. The findings revealed that at specific concentrations, the compound exhibited reduced cell viability, suggesting potential applications in cancer therapy .
Comparative Analysis
Compound | Molecular Weight | Antidepressant Activity | Cytotoxicity |
---|---|---|---|
2-(4-Fluorophenyl)azepane | 201.26 g/mol | Yes | Moderate |
2-Phenylazepane | 185.25 g/mol | Yes | Low |
2-(4-Chlorophenyl)azepane | 201.26 g/mol | Moderate | High |
Propiedades
IUPAC Name |
2-(4-fluorophenyl)azepane |
Source
|
---|---|---|
Source | PubChem | |
URL | https://pubchem.ncbi.nlm.nih.gov | |
Description | Data deposited in or computed by PubChem | |
InChI |
InChI=1S/C12H16FN/c13-11-7-5-10(6-8-11)12-4-2-1-3-9-14-12/h5-8,12,14H,1-4,9H2 |
Source
|
Source | PubChem | |
URL | https://pubchem.ncbi.nlm.nih.gov | |
Description | Data deposited in or computed by PubChem | |
InChI Key |
KYRNAJNHLFLGFP-UHFFFAOYSA-N |
Source
|
Source | PubChem | |
URL | https://pubchem.ncbi.nlm.nih.gov | |
Description | Data deposited in or computed by PubChem | |
Canonical SMILES |
C1CCC(NCC1)C2=CC=C(C=C2)F |
Source
|
Source | PubChem | |
URL | https://pubchem.ncbi.nlm.nih.gov | |
Description | Data deposited in or computed by PubChem | |
Molecular Formula |
C12H16FN |
Source
|
Source | PubChem | |
URL | https://pubchem.ncbi.nlm.nih.gov | |
Description | Data deposited in or computed by PubChem | |
DSSTOX Substance ID |
DTXSID90394283 |
Source
|
Record name | 2-(4-fluorophenyl)azepane | |
Source | EPA DSSTox | |
URL | https://comptox.epa.gov/dashboard/DTXSID90394283 | |
Description | DSSTox provides a high quality public chemistry resource for supporting improved predictive toxicology. | |
Molecular Weight |
193.26 g/mol |
Source
|
Source | PubChem | |
URL | https://pubchem.ncbi.nlm.nih.gov | |
Description | Data deposited in or computed by PubChem | |
CAS No. |
168890-44-6 |
Source
|
Record name | 2-(4-fluorophenyl)azepane | |
Source | EPA DSSTox | |
URL | https://comptox.epa.gov/dashboard/DTXSID90394283 | |
Description | DSSTox provides a high quality public chemistry resource for supporting improved predictive toxicology. | |
Descargo de responsabilidad e información sobre productos de investigación in vitro
Tenga en cuenta que todos los artículos e información de productos presentados en BenchChem están destinados únicamente con fines informativos. Los productos disponibles para la compra en BenchChem están diseñados específicamente para estudios in vitro, que se realizan fuera de organismos vivos. Los estudios in vitro, derivados del término latino "in vidrio", involucran experimentos realizados en entornos de laboratorio controlados utilizando células o tejidos. Es importante tener en cuenta que estos productos no se clasifican como medicamentos y no han recibido la aprobación de la FDA para la prevención, tratamiento o cura de ninguna condición médica, dolencia o enfermedad. Debemos enfatizar que cualquier forma de introducción corporal de estos productos en humanos o animales está estrictamente prohibida por ley. Es esencial adherirse a estas pautas para garantizar el cumplimiento de los estándares legales y éticos en la investigación y experimentación.