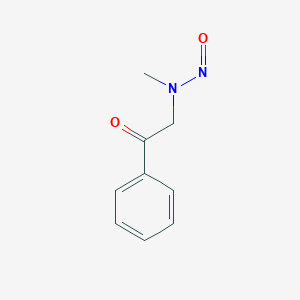
Endostatin
Descripción general
Descripción
Endostatin, a 20 kDa C-terminal proteolytic fragment of collagen XVIII, is a potent endogenous angiogenesis inhibitor. It suppresses endothelial cell proliferation, migration, and survival by modulating focal adhesion kinase (FAK), paxillin phosphorylation, and actin cytoskeletal dynamics . Recombinant this compound has been produced in yeast (10–20 mg/L yield) and Escherichia coli (150 mg/L yield), with both forms demonstrating antiangiogenic and antitumor efficacy in preclinical models, including renal cell carcinoma and non-small cell lung cancer (NSCLC) . Its mechanisms include inhibition of matrix metalloproteinase-2 (MMP-2) activation, disruption of integrin α5β1/caveolin-1 signaling, and induction of endothelial cell apoptosis .
Métodos De Preparación
Rutas sintéticas y condiciones de reacción: La endostatina se puede producir a través de la tecnología de ADN recombinante. El gen que codifica la endostatina se inserta en un vector de expresión adecuado, que luego se introduce en una célula huésped, como Escherichia coli o levadura. Las células huésped se cultivan en condiciones específicas para expresar la proteína endostatina, que luego se purifica utilizando técnicas como la cromatografía de afinidad .
Métodos de producción industrial: En entornos industriales, la producción a gran escala de endostatina implica el uso de biorreactores para cultivar las células huésped. El proceso incluye pasos de fermentación, lisis celular y purificación de proteínas. La variante de endostatina humana recombinante (rh-Es) ha sido aprobada para su uso en el tratamiento del cáncer de pulmón de células no pequeñas en China desde 2006 .
Análisis De Reacciones Químicas
ATP Hydrolysis Activity
Endostatin demonstrates intrinsic ATPase activity, hydrolyzing ATP to ADP and inorganic phosphate. This activity is pH-dependent, with optimal hydrolysis observed at pH 6.0–7.0 . Mutagenesis studies reveal that ATPase activity correlates with antiangiogenic efficacy:
- E-M mutant : 2.5× higher ATPase activity than wild-type (WT), leading to enhanced inhibition of endothelial cell proliferation (IC₅₀: 0.28 μM vs. WT’s 0.45 μM) .
- K96A/K96R/E176A mutants : Reduced ATPase activity (50–70% of WT) and diminished antitumor effects .
Mechanistic Impact : ATP hydrolysis modulates intracellular ATP levels, regulating endothelial cell apoptosis via Erk/p38/Src phosphorylation pathways .
Zinc Binding and Structural Stabilization
This compound binds zinc via three N-terminal histidine residues (His1, His3, His11) . While zinc is not essential for antiangiogenic activity, it stabilizes the protein:
- H5 mutant (lacking zinc-binding residues): Retains antiangiogenic activity but shows reduced thermal stability (denaturation at 45°C vs. WT’s 60°C) .
- Zinc-bound this compound : Resists proteolysis and maintains structural integrity under denaturing conditions .
Functional Role : Zinc facilitates multimerization, enhancing binding to fibroblast growth factor-2 (FGF-2) in SPR assays .
pH-Dependent Activity and Nanoparticle Release
This compound’s activity and release kinetics are influenced by pH:
Nanoparticle Component | pH 7.4 (Release) | pH 6.8 (Release) | Cytotoxicity at 250 μg/mL |
---|---|---|---|
CHT-g-PEI-PEG-NH₂ | 25% over 72 hrs | 80% over 72 hrs | 13.36% cell death |
Acidic tumor microenvironments (pH 6.8) enhance nanoparticle swelling, releasing this compound to inhibit squamous cell carcinoma .
Peptide Fragments and Mutagenesis
Proteolytic processing generates bioactive fragments, with distinct regions mediating antiangiogenic effects:
Notable Mutants :
- P125A-endostatin : 2× higher endothelial cell inhibition (IC₅₀: 0.2 μM vs. WT’s 0.4 μM) .
- mEP-AA (cysteine substitutions): Loses VEGFR-3 binding entirely .
Receptor Binding and Signal Inhibition
This compound competitively inhibits growth factor-receptor interactions through specific epitopes:
Mechanistic Insight :
Aplicaciones Científicas De Investigación
La endostatina tiene una amplia gama de aplicaciones en la investigación científica, particularmente en los campos de la química, la biología, la medicina y la industria.
Química: En química, la endostatina se estudia por sus interacciones con varios receptores y su papel en la inhibición de la angiogénesis .
Biología: En la investigación biológica, la endostatina se utiliza para estudiar los mecanismos de la angiogénesis y el papel de los componentes de la matriz extracelular en la regulación de la formación de vasos sanguíneos .
Medicina: La endostatina se investiga ampliamente por su potencial en la terapia contra el cáncer. Se ha demostrado que inhibe el crecimiento de tumores primarios y metástasis en modelos animales . Los ensayos clínicos han demostrado su eficacia en el tratamiento del cáncer de pulmón de células no pequeñas y otras malignidades .
Industria: En la industria farmacéutica, la endostatina se utiliza para desarrollar fármacos contra el cáncer. Su forma recombinante, rh-Es, se utiliza en combinación con otras terapias para mejorar la eficacia del tratamiento .
Mecanismo De Acción
La endostatina ejerce sus efectos inhibiendo la angiogénesis a través de múltiples vías. Se une a varios receptores, como los receptores del factor de crecimiento endotelial vascular (VEGFR-2 y VEGFR-3), glipicano 1 y 4, e integrina α5β1 . Esta unión interfiere con las vías de señalización que promueven la formación de vasos sanguíneos, lo que lleva a la inhibición de la proliferación, la migración y la supervivencia de las células endoteliales . La endostatina también induce la apoptosis en las células endoteliales al inhibir la ciclina D1 y al interrumpir las adhesiones célula-matriz .
Comparación Con Compuestos Similares
Mechanistic Comparison with Angiostatin
Angiostatin, a kringle 1-4 fragment of plasminogen, shares antiangiogenic properties with endostatin but differs in molecular targets and mechanisms. While this compound inhibits MMP-2 and integrin-mediated signaling, angiostatin binds ATP synthase and annexin II, suppressing endothelial cell proliferation . In ovarian cancer xenografts, angiostatin monotherapy (20 mg/kg/day) achieved 68% tumor suppression, outperforming this compound (50% suppression at 10 mg/kg/day). However, their combination synergistically reduced tumor growth by 92%, highlighting complementary pathways .
Engineered Variants: Enhanced Activity of E-M
E-M, an engineered this compound with 3.5-fold higher ATPase activity, exhibits superior anti-tumor effects compared to wild-type this compound. In NSCLC models, E-M (1 mg/kg/day) reduced tumor-associated macrophage (TAM) infiltration by 60% and microvessel density by 45%, outperforming wild-type this compound (30% and 25%, respectively) . E-M’s efficacy stems from enhanced binding to nucleolin and integrin α5β1 on M2 macrophages, blocking p38 MAPK/Erk1/2 pathways and TAM polarization .
Functional Peptide Fragments: The Case of E4
The C-terminal peptide E4 (residues 158–178 of this compound) retains antifibrotic activity but lacks antiangiogenic effects. In bleomycin-induced pulmonary fibrosis, E4 (10 µM) reduced lysyl oxidase (LOX) and Egr-1 expression by 70%, reversing collagen deposition . In contrast, the N-terminal domain of this compound is critical for MMP-2 inhibition and endothelial apoptosis, underscoring functional segregation within the protein .
Cross-talk with Small Molecule Inhibitors: ACS-1
ACS-1, a dithiolethione compound, mimics this compound’s activation of protein phosphatase 2A (PP2A) but targets the Akt pathway. Both agents inhibit ERK1/2 signaling, but ACS-1 (10 µM) achieves 50% PP2A activation within 1 hour, whereas this compound requires prolonged exposure . This divergence suggests distinct upstream regulators, positioning ACS-1 as a complementary small-molecule agent.
Synergistic Effects in Antiangiogenic Therapy
Combining this compound with angiostatin or chemotherapy enhances efficacy. In ovarian cancer, co-administration of this compound (5 mg/kg) and angiostatin (5 mg/kg) reduced tumor volume by 92%, compared to 68% (angiostatin alone) and 50% (this compound alone) . Similarly, continuous this compound infusion via osmotic pump (5 mg/kg/day) achieved 80% tumor regression in pancreatic cancer, surpassing bolus dosing (50% regression at 10 mg/kg/day) .
Comparative Efficacy in Disease Models
Actividad Biológica
Endostatin, a proteolytic fragment of collagen XVIII, is recognized for its potent antiangiogenic and antitumor properties. This article delves into the biological activity of this compound, focusing on its mechanisms of action, effects on endothelial cells, and clinical implications.
This compound exerts its biological effects primarily by inhibiting angiogenesis—the formation of new blood vessels from pre-existing ones. This process is crucial for tumor growth and metastasis. The mechanisms through which this compound mediates its effects include:
- Inhibition of Endothelial Cell Proliferation and Migration : this compound significantly reduces the proliferation and migration of endothelial cells in vitro. Studies have demonstrated that it can inhibit tube formation and induce apoptosis in these cells by activating various signaling pathways, including the MAPK pathway through integrin binding .
- ATPase Activity : Recent research has uncovered that this compound possesses intrinsic ATPase activity, which correlates positively with its antiangiogenic effects. Mutants of this compound with enhanced ATPase activity displayed greater inhibition of endothelial cell functions and tumor growth compared to wild-type this compound .
- Binding to Cell Surface Receptors : this compound interacts with several receptors on endothelial cells, including integrins and glypicans. This binding initiates various intracellular signaling cascades that lead to reduced cell migration and proliferation .
In Vivo Effects
This compound's efficacy has been demonstrated in several in vivo models:
- Angiogenesis Models : In a surrogate model using human endothelial cells implanted in immunodeficient mice, systemic delivery of recombinant human this compound resulted in a dramatic reduction in vessel formation (95% inhibition) after 20 days. It effectively hampered the recruitment of perivascular cells, further supporting its role as an antiangiogenic agent .
- Tumor Growth Inhibition : this compound has shown significant antitumor activity in various cancer models. For instance, a study involving human ovarian cancer indicated that a mutant form of this compound (P125A-endostatin) exhibited superior inhibitory effects on tumor growth compared to native this compound .
Case Studies
Several case studies highlight the therapeutic potential of this compound in clinical settings:
- Combination Therapy : A recent case report illustrated the effectiveness of recombinant human this compound combined with chemotherapy in a patient with epidermal growth factor receptor-negative miliary lung adenocarcinoma. The treatment led to significant tumor response, showcasing this compound's potential in enhancing chemotherapy efficacy .
- Kidney Fibrosis : Research has explored the role of this compound in kidney fibrosis, particularly in aging mice. Treatment with this compound resulted in delayed recovery from femoral artery ligation and increased renal fibrosis markers, suggesting that while it inhibits angiogenesis, it may also contribute to adverse effects under certain conditions .
Data Summary
The following table summarizes key findings related to the biological activity of this compound:
Q & A
Q. (Basic) What experimental models are commonly used to study Endostatin’s anti-angiogenic activity?
This compound’s anti-angiogenic effects are typically evaluated using human umbilical vein endothelial cells (HUVECs) for in vitro migration assays (e.g., VEGF-induced migration inhibition studies) . In vivo, apolipoprotein E-deficient (apoE -/-) mice are a key model for atherosclerosis research, where this compound reduces intimal neovascularization and plaque growth . For tumor studies, subcutaneous xenografts in nude mice (e.g., human renal cell carcinoma) are employed to assess tumor suppression . Methodologically, ensure matched controls (e.g., buffer-treated groups) and standardized dosing (e.g., 20 mg/kg/day for mice) to minimize variability .
Q. (Basic) What biochemical methods are used to quantify this compound expression and localization?
ELISA and Western blot are standard for quantifying this compound in conditioned media or tissue lysates . Antibody validation is critical: use monoclonal antibodies with confirmed specificity for human/mouse this compound (e.g., Rabbit anti-Endostatin for WB) . For localization, immunohistochemistry on tissue sections (e.g., aortic plaques in mice) can visualize intimal neovascularization inhibition . Include controls like plasmid-free constructs and baseline secretion levels (e.g., MSC-seeded scaffolds in normoxic vs. hypoxic conditions) to contextualize results .
Q. (Basic) How does this compound induce endothelial cell cycle arrest?
This compound downregulates cyclin D1 via transcriptional repression of its promoter, specifically targeting the LEF1 binding site . Methodologically, use promoter deletion constructs and β-catenin overexpression to validate LEF1-dependent repression . Flow cytometry can confirm G1 arrest by assessing hyperphosphorylated retinoblastoma protein (Rb) levels. Note that cyclin D1 overexpression rescues this compound-induced arrest, necessitating siRNA knockdown controls .
Q. (Advanced) How can researchers resolve contradictions in this compound’s reported molecular targets (e.g., integrins vs. heparan sulfate)?
Contradictions arise from overlapping binding sites (e.g., Arg27/Arg139 residues for both integrins and heparin) . Use surface plasmon resonance (SPR) to quantify binding affinities (e.g., KD = ~1.8 × 10⁻⁸ M for α5β1 integrin) and site-directed mutagenesis to isolate critical residues . Competitive assays (e.g., heparin pre-incubation) can determine if integrin-heparan sulfate interactions are mutually exclusive . Molecular modeling of the αvβ3 integrin-endostatin interface further clarifies steric constraints .
Q. (Advanced) What experimental design considerations are critical for studies on this compound production in 3D constructs?
Multifactorial ANOVA (e.g., 5-factor: oxygen tension, scaffold material, cell type, plasmid load, collection period) is essential to dissect variables affecting this compound secretion . For chondrogenic constructs, optimize plasmid load (e.g., 20 µg vs. 0 µg) and scaffold type (e.g., collagen I/III vs. II) under controlled oxygen gradients (21% vs. 5%) . Include power analysis (e.g., power = 1 for large effects) and post hoc tests (Fisher’s PLSD) to ensure statistical rigor .
Q. (Advanced) How can pharmacokinetic modeling improve this compound’s therapeutic application?
Sensitivity analysis of parameters like drug clearance and tumor growth rates can identify optimal dosing schedules . For example, compare this compound’s efficacy to Bevacizumab using pharmacokinetic data (e.g., doses normalized to 4–8 mg/kg/day) . Use compartmental models to simulate prolonged treatment effects, ensuring alignment with in vivo outcomes (e.g., 85% plaque growth inhibition in mice) .
Q. (Advanced) What challenges arise when translating preclinical this compound findings to clinical trials?
Clinical trials face discordance in response metrics (e.g., tumor shrinkage vs. angiogenesis inhibition) and unclear mechanisms . Preclinically, prioritize biomarker validation (e.g., intimal vessel density in plaques) . For trials, adopt adaptive designs that monitor surrogate endpoints (e.g., circulating this compound levels) and combine with VEGF inhibitors to address resistance . Note historical pitfalls: early trials failed due to inappropriate outcome measures, not efficacy .
Q. (Advanced) How can transcriptional regulation studies elucidate this compound’s anti-angiogenic mechanisms?
Use cyclin D1 promoter reporter assays with serial deletions to identify responsive elements (e.g., LEF1 site) . Co-transfect β-catenin to test pathway crosstalk, and employ inhibitors (e.g., Wnt/β-catenin blockers) to confirm specificity . Chromatin immunoprecipitation (ChIP) can map this compound-induced changes in transcription factor binding (e.g., TCF/LEF1) at target promoters.
Q. (Advanced) How to address discrepancies between this compound’s preclinical efficacy and clinical outcomes?
Reconcile differences by re-evaluating dosing protocols (e.g., continuous infusion vs. bolus) and patient stratification (e.g., tumors with high integrin αvβ3 expression) . Preclinical models should mimic human pharmacokinetics (e.g., sustained serum levels >100 ng/mL) . Clinically, incorporate functional imaging (e.g., dynamic contrast-enhanced MRI) to quantify angiogenesis inhibition directly .
Q. (Advanced) What methodologies validate this compound’s anti-angiogenic mechanisms across models?
Combine functional assays (e.g., HUVEC migration, Matrigel tube formation) with genetic knockdown (e.g., integrin α5 siRNA) to confirm target specificity . In vivo, correlate this compound levels with neovascularization markers (e.g., CD31⁺ vessels in plaques) . Use meta-analysis of cross-study data (e.g., comparing TNP-470 and this compound in Hahnfeldt’s models) to identify conserved pathways .
Propiedades
IUPAC Name |
N-methyl-N-phenacylnitrous amide | |
---|---|---|
Source | PubChem | |
URL | https://pubchem.ncbi.nlm.nih.gov | |
Description | Data deposited in or computed by PubChem | |
InChI |
InChI=1S/C9H10N2O2/c1-11(10-13)7-9(12)8-5-3-2-4-6-8/h2-6H,7H2,1H3 | |
Source | PubChem | |
URL | https://pubchem.ncbi.nlm.nih.gov | |
Description | Data deposited in or computed by PubChem | |
InChI Key |
AAFYOVPTFNNVDN-UHFFFAOYSA-N | |
Source | PubChem | |
URL | https://pubchem.ncbi.nlm.nih.gov | |
Description | Data deposited in or computed by PubChem | |
Canonical SMILES |
CN(CC(=O)C1=CC=CC=C1)N=O | |
Source | PubChem | |
URL | https://pubchem.ncbi.nlm.nih.gov | |
Description | Data deposited in or computed by PubChem | |
Molecular Formula |
C9H10N2O2 | |
Source | PubChem | |
URL | https://pubchem.ncbi.nlm.nih.gov | |
Description | Data deposited in or computed by PubChem | |
DSSTOX Substance ID |
DTXSID10204557 | |
Record name | Ethanone, 2-(methylnitrosoamino)-1-phenyl- | |
Source | EPA DSSTox | |
URL | https://comptox.epa.gov/dashboard/DTXSID10204557 | |
Description | DSSTox provides a high quality public chemistry resource for supporting improved predictive toxicology. | |
Molecular Weight |
178.19 g/mol | |
Source | PubChem | |
URL | https://pubchem.ncbi.nlm.nih.gov | |
Description | Data deposited in or computed by PubChem | |
CAS No. |
55984-52-6, 187888-07-9 | |
Record name | Ethanone, 2-(methylnitrosoamino)-1-phenyl- | |
Source | ChemIDplus | |
URL | https://pubchem.ncbi.nlm.nih.gov/substance/?source=chemidplus&sourceid=0055984526 | |
Description | ChemIDplus is a free, web search system that provides access to the structure and nomenclature authority files used for the identification of chemical substances cited in National Library of Medicine (NLM) databases, including the TOXNET system. | |
Record name | Endostatin | |
Source | DrugBank | |
URL | https://www.drugbank.ca/drugs/DB06423 | |
Description | The DrugBank database is a unique bioinformatics and cheminformatics resource that combines detailed drug (i.e. chemical, pharmacological and pharmaceutical) data with comprehensive drug target (i.e. sequence, structure, and pathway) information. | |
Explanation | Creative Common's Attribution-NonCommercial 4.0 International License (http://creativecommons.org/licenses/by-nc/4.0/legalcode) | |
Record name | Ethanone, 2-(methylnitrosoamino)-1-phenyl- | |
Source | EPA DSSTox | |
URL | https://comptox.epa.gov/dashboard/DTXSID10204557 | |
Description | DSSTox provides a high quality public chemistry resource for supporting improved predictive toxicology. | |
Retrosynthesis Analysis
AI-Powered Synthesis Planning: Our tool employs the Template_relevance Pistachio, Template_relevance Bkms_metabolic, Template_relevance Pistachio_ringbreaker, Template_relevance Reaxys, Template_relevance Reaxys_biocatalysis model, leveraging a vast database of chemical reactions to predict feasible synthetic routes.
One-Step Synthesis Focus: Specifically designed for one-step synthesis, it provides concise and direct routes for your target compounds, streamlining the synthesis process.
Accurate Predictions: Utilizing the extensive PISTACHIO, BKMS_METABOLIC, PISTACHIO_RINGBREAKER, REAXYS, REAXYS_BIOCATALYSIS database, our tool offers high-accuracy predictions, reflecting the latest in chemical research and data.
Strategy Settings
Precursor scoring | Relevance Heuristic |
---|---|
Min. plausibility | 0.01 |
Model | Template_relevance |
Template Set | Pistachio/Bkms_metabolic/Pistachio_ringbreaker/Reaxys/Reaxys_biocatalysis |
Top-N result to add to graph | 6 |
Feasible Synthetic Routes
Descargo de responsabilidad e información sobre productos de investigación in vitro
Tenga en cuenta que todos los artículos e información de productos presentados en BenchChem están destinados únicamente con fines informativos. Los productos disponibles para la compra en BenchChem están diseñados específicamente para estudios in vitro, que se realizan fuera de organismos vivos. Los estudios in vitro, derivados del término latino "in vidrio", involucran experimentos realizados en entornos de laboratorio controlados utilizando células o tejidos. Es importante tener en cuenta que estos productos no se clasifican como medicamentos y no han recibido la aprobación de la FDA para la prevención, tratamiento o cura de ninguna condición médica, dolencia o enfermedad. Debemos enfatizar que cualquier forma de introducción corporal de estos productos en humanos o animales está estrictamente prohibida por ley. Es esencial adherirse a estas pautas para garantizar el cumplimiento de los estándares legales y éticos en la investigación y experimentación.