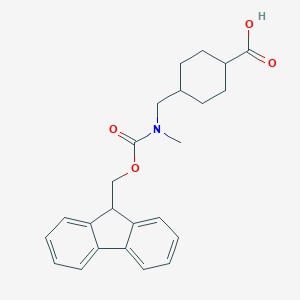
Fmoc-N-methyl-tranexamic acid
- Haga clic en CONSULTA RÁPIDA para recibir una cotización de nuestro equipo de expertos.
- Con productos de calidad a un precio COMPETITIVO, puede centrarse más en su investigación.
Descripción general
Descripción
Fmoc-N-methyl-tranexamic acid: is a derivative of tranexamic acid, which is a synthetic lysine analog. This compound is characterized by the presence of a fluorenylmethyloxycarbonyl (Fmoc) group, which is commonly used in peptide synthesis as a protecting group for amino acids. The addition of the N-methyl group enhances the compound’s stability and bioavailability, making it a valuable tool in various scientific research applications .
Métodos De Preparación
Synthetic Routes and Reaction Conditions: The synthesis of Fmoc-N-methyl-tranexamic acid typically involves the use of solid-phase peptide synthesis (SPPS) techniques. One common method employs 2-chlorotrityl chloride (2-CTC) resin as a temporary protective group for the carboxylic acid. The N-methylation step can be achieved using either dimethyl sulfate or methyl iodide in the Biron-Kessler method . The desired amino acids, such as Fmoc-N-methyl-threonine and Fmoc-N-methyl-beta-alanine, are synthesized with high yield and purity using these strategies .
Industrial Production Methods: Industrial production of this compound involves large-scale SPPS techniques, utilizing automated peptide synthesizers to ensure high efficiency and consistency. The process includes the sequential addition of protected amino acids to a growing peptide chain anchored to a solid support, followed by deprotection and cleavage steps to obtain the final product .
Análisis De Reacciones Químicas
Types of Reactions: Fmoc-N-methyl-tranexamic acid undergoes various chemical reactions, including:
Substitution Reactions: The Fmoc group can be removed under basic conditions, typically using piperidine, to expose the free amine group for further functionalization.
Oxidation and Reduction Reactions: The compound can participate in oxidation and reduction reactions, depending on the specific functional groups present in the molecule.
Common Reagents and Conditions:
Substitution Reactions: Piperidine is commonly used for Fmoc group removal.
Oxidation and Reduction Reactions: Standard oxidizing and reducing agents, such as hydrogen peroxide and sodium borohydride, can be employed.
Major Products Formed: The major products formed from these reactions include deprotected amino acids and various functionalized derivatives, depending on the specific reagents and conditions used .
Aplicaciones Científicas De Investigación
Chemistry: Fmoc-N-methyl-tranexamic acid is widely used in peptide synthesis as a building block for the preparation of N-methylated peptides, which have enhanced stability and bioavailability .
Biology: In biological research, this compound is utilized to study protein-protein interactions and enzyme-substrate specificity, owing to its ability to mimic natural amino acids .
Medicine: this compound has potential therapeutic applications, particularly in the development of peptide-based drugs with improved pharmacokinetic properties .
Industry: In the industrial sector, this compound is employed in the production of specialized biomaterials and hydrogels for various applications .
Mecanismo De Acción
The mechanism of action of Fmoc-N-methyl-tranexamic acid involves its role as a protecting group in peptide synthesis. The Fmoc group protects the amino group of the amino acid during the synthesis process, preventing unwanted side reactions. Upon completion of the synthesis, the Fmoc group is removed under basic conditions to expose the free amine group for further functionalization . The N-methyl group enhances the stability and bioavailability of the resulting peptides, making them more resistant to enzymatic degradation .
Comparación Con Compuestos Similares
Fmoc-tranexamic acid: Similar to Fmoc-N-methyl-tranexamic acid but lacks the N-methyl group, resulting in lower stability and bioavailability.
Fmoc-N-methyl-threonine: Another N-methylated amino acid used in peptide synthesis, offering similar stability and bioavailability benefits.
Fmoc-N-methyl-beta-alanine: Used in peptide synthesis with enhanced stability and bioavailability.
Uniqueness: this compound stands out due to its combination of the Fmoc protecting group and the N-methyl group, which together provide enhanced stability, bioavailability, and resistance to enzymatic degradation. This makes it a valuable tool in the synthesis of peptide-based drugs and biomaterials .
Actividad Biológica
Fmoc-N-methyl-tranexamic acid (Fmoc-NMTA) is a derivative of tranexamic acid (TA), which is primarily known for its antifibrinolytic properties. The addition of the Fmoc (9-Fluorenylmethoxycarbonyl) group enhances the compound's stability and bioavailability, making it a valuable tool in peptide synthesis and biological research. This article explores the biological activity of Fmoc-NMTA, focusing on its mechanisms, applications, and relevant case studies.
This compound has the molecular formula C24H27NO4 and features both an N-methyl group and an Fmoc protecting group. These modifications contribute to its unique properties compared to standard tranexamic acid.
Property | Value |
---|---|
Molecular Formula | C24H27NO4 |
Molecular Weight | 393.48 g/mol |
Solubility | Soluble in DMF |
Stability | High under acidic conditions, labile under basic conditions |
Fmoc-NMTA functions primarily as a lysine analog, inhibiting fibrinolysis by blocking plasminogen activation. This action is crucial in various therapeutic contexts, particularly in managing bleeding disorders. Additionally, its structural features allow it to mimic natural amino acids, facilitating studies on protein interactions and enzyme specificity.
Key Mechanisms:
- Inhibition of Plasminogen Activation : Fmoc-NMTA competes with lysine for binding sites on plasminogen activators.
- Protein-Protein Interactions : The compound is utilized in research to dissect interactions between proteins due to its ability to mimic natural amino acids.
- Enzyme-Substrate Specificity : It aids in studying how enzymes interact with substrates in biochemical pathways.
Biological Activity
Recent studies have highlighted the potential anticancer properties of tranexamic acid and its derivatives, including Fmoc-NMTA. Research indicates that these compounds can reduce cancer cell viability through several mechanisms:
- Cell Viability Reduction : In vitro studies demonstrate that Fmoc-NMTA can suppress the viability of various cancer cell lines, including melanoma and breast cancer cells.
- Inhibition of Tumor Growth : Animal models have shown that treatment with Fmoc-NMTA can lead to reduced tumor growth rates.
- Alteration of Signaling Pathways : The compound has been shown to affect intracellular signaling pathways that regulate cell proliferation and oncogenic transformation.
Case Study 1: Anticancer Activity
A study demonstrated that Fmoc-NMTA significantly reduced the viability of MDA-MB-468 breast cancer cells in vitro. The reduction was concentration-dependent, with notable effects observed at low concentrations (0.25% w/v). The mechanism involved inhibition of DNA synthesis and interference with oncogenic signaling pathways such as MYC overexpression.
Case Study 2: Treatment of Chronic Subdural Hematoma (CSDH)
In a clinical case report involving an 86-year-old patient treated with tranexamic acid for CSDH, complete resolution was achieved after one month of therapy without recurrence over two years. This suggests that similar derivatives like Fmoc-NMTA may hold promise in conservative management strategies for hematomas by reducing hyper-fibrinolytic activity.
Research Findings
Research findings indicate that Fmoc-NMTA not only retains the antifibrinolytic properties of tranexamic acid but also enhances the stability and bioavailability necessary for effective therapeutic applications.
Summary of Research Findings:
- Cell Lines Tested : Melanoma (B16), breast (BT474), prostate (DU145).
- Viability Assays : MTT and XTT assays showed significant reductions in cell viability across multiple cancer types.
- Mechanistic Insights : Inhibition of protein synthesis and modification of acetylation status were observed as key biochemical actions.
Propiedades
Número CAS |
173690-50-1 |
---|---|
Fórmula molecular |
C24H27NO4 |
Peso molecular |
393.5 g/mol |
Nombre IUPAC |
4-[[[2-(9H-fluoren-9-ylmethoxy)-2-oxoethyl]amino]methyl]cyclohexane-1-carboxylic acid |
InChI |
InChI=1S/C24H27NO4/c26-23(14-25-13-16-9-11-17(12-10-16)24(27)28)29-15-22-20-7-3-1-5-18(20)19-6-2-4-8-21(19)22/h1-8,16-17,22,25H,9-15H2,(H,27,28) |
Clave InChI |
IPLFYSZHICEXRE-UHFFFAOYSA-N |
SMILES |
CN(CC1CCC(CC1)C(=O)O)C(=O)OCC2C3=CC=CC=C3C4=CC=CC=C24 |
SMILES canónico |
C1CC(CCC1CNCC(=O)OCC2C3=CC=CC=C3C4=CC=CC=C24)C(=O)O |
Origen del producto |
United States |
Descargo de responsabilidad e información sobre productos de investigación in vitro
Tenga en cuenta que todos los artículos e información de productos presentados en BenchChem están destinados únicamente con fines informativos. Los productos disponibles para la compra en BenchChem están diseñados específicamente para estudios in vitro, que se realizan fuera de organismos vivos. Los estudios in vitro, derivados del término latino "in vidrio", involucran experimentos realizados en entornos de laboratorio controlados utilizando células o tejidos. Es importante tener en cuenta que estos productos no se clasifican como medicamentos y no han recibido la aprobación de la FDA para la prevención, tratamiento o cura de ninguna condición médica, dolencia o enfermedad. Debemos enfatizar que cualquier forma de introducción corporal de estos productos en humanos o animales está estrictamente prohibida por ley. Es esencial adherirse a estas pautas para garantizar el cumplimiento de los estándares legales y éticos en la investigación y experimentación.