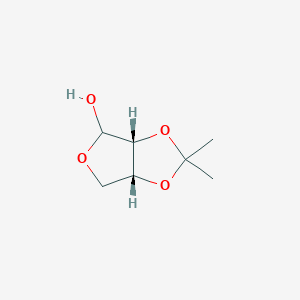
2,3-O-Isopropylidene-D-erythrofuranose
Descripción general
Descripción
2,3-O-Isopropylidene-D-erythrofuranose (CAS: 189996-60-9) is a protected sugar derivative widely used in organic synthesis. Its molecular formula is C₇H₁₂O₄, with a molecular weight of 160.17 g/mol . The compound is characterized by a fused bicyclic structure formed by the 2,3-O-isopropylidene acetal protecting group on the D-erythrofuranose backbone. This protection enhances stability and directs regioselectivity in subsequent reactions.
Métodos De Preparación
Acetonation of D-Erythrose Using Acidic Acetone
The most widely documented method involves the acetonation of D-erythrose with acetone in the presence of an acid catalyst. This approach parallels procedures developed for analogous sugars, such as D-lyxose .
Reaction Conditions and Optimization
The reaction employs anhydrous acetone , cupric sulfate (as a desiccant), and concentrated sulfuric acid (catalyst). Key modifications to classical acetonation protocols include:
-
Magnetic stirring to maintain homogeneity and temperature control .
-
Extended reaction times (5 days) at 35°C to maximize yield .
-
Post-reaction neutralization with anion-exchange resin to remove acidic byproducts .
A typical protocol involves:
-
Dissolving 20 g of D-erythrose in 500 mL of acetone.
-
Adding 50 g of anhydrous CuSO₄ and 0.5 mL of H₂SO₄.
-
Stirring at 35°C for 5 days.
-
Filtering, resin treatment, and vacuum distillation to isolate the product .
Table 1: Yield Data for Acetonation of D-Erythrose
The product is purified via crystallization from toluene, yielding a crystalline solid with a melting point of 81.5–82.5°C .
Thiazole Intermediate-Based Synthesis
An alternative route utilizes thiazole intermediates to construct the furanose ring, as hinted at in industrial synthesis protocols . While detailed mechanisms remain proprietary, the general steps include:
Key Steps
-
Precursor Activation : Thiazole derivatives are functionalized to introduce hydroxyl and isopropylidene groups.
-
Cyclization : Acid-catalyzed cyclization forms the furanose ring.
-
Deprotection : Selective removal of auxiliary protecting groups yields the target compound .
This method is favored for scalability but requires stringent control over stereochemistry to avoid epimerization .
Bromine Oxidation of Protected Derivatives
Bromine-mediated oxidation offers a pathway to this compound from its alcohol precursors. A representative procedure involves:
Protocol Overview
-
Treating 0.19 g of 2,3-O-isopropylidene-D-erythritol with barium bromide and bromine in hydrobromic acid at 0°C .
-
Quenching with ethylene to remove excess bromine.
This method achieves moderate yields (≈84%) but is critical for accessing oxidized derivatives .
Borohydride Reduction of Keto Intermediates
Sodium borohydride reduction of keto-protected intermediates provides a high-yield route:
Procedure
-
Dissolving 1.0 g of 2,3-O-isopropylidene-D-erythrulose in 5 mL of water.
-
Adding 0.175 g of NaBH₄ at 0°C.
-
Stirring overnight, followed by cation-exchange resin treatment and solvent evaporation .
Table 2: Reduction Efficiency
The product crystallizes from ethanol-pentane mixtures, demonstrating the method’s reliability .
Diacyl Derivative Synthesis
Post-acetonation, the compound undergoes further functionalization to produce diesters, expanding its synthetic utility:
Diacetate and Dibenzoate Formation
-
Diacetate Synthesis : Treating this compound with acetic anhydride in pyridine yields the 1,5-diacetyl derivative .
-
Dibenzoate Synthesis : Similarly, benzoyl chloride in pyridine produces the 1,5-dibenzoyl ester .
Both reactions proceed in >90% yield, underscoring their practicality for introducing acyl groups .
Comparative Analysis of Methods
Table 3: Method Comparison
Method | Advantages | Limitations |
---|---|---|
Acidic Acetonation | High stereochemical control | Long reaction time (5 days) |
Thiazole Intermediate | Scalability | Proprietary details unavailable |
Bromine Oxidation | Access to oxidized derivatives | Requires toxic reagents |
Borohydride Reduction | High yield (80%) | Limited to keto precursors |
Diacyl Formation | Versatile functionalization | Requires anhydrous conditions |
Análisis De Reacciones Químicas
2,3-O-Isopropylidene-D-erythrofuranose undergoes various chemical reactions, including oxidation, reduction, and substitution. Common reagents used in these reactions include silyl ketene acetals and other organic solvents . The major products formed from these reactions are often intermediates used in the synthesis of more complex molecules .
Aplicaciones Científicas De Investigación
Introduction to 2,3-O-Isopropylidene-D-erythrofuranose
This compound is a carbohydrate derivative that plays a significant role in various scientific research applications. Its unique structural properties make it a valuable compound in organic chemistry, particularly in the synthesis of glycosides and as a building block in the development of pharmaceuticals. This article explores its applications, providing comprehensive data and case studies that highlight its relevance in scientific research.
Synthesis of Glycosides
This compound is widely used as a glycosyl donor in the synthesis of various glycosides. Its ability to protect hydroxyl groups while facilitating glycosylation reactions makes it an essential intermediate in carbohydrate chemistry. For instance, researchers have utilized this compound to generate glycoside mimics that exhibit biological activity relevant to drug development .
Anticancer Research
Recent studies have indicated that derivatives of this compound exhibit anticancer properties by inhibiting protein synthesis through interactions with cysteine residues in proteins. This mechanism positions it as a potential lead compound for developing new anticancer agents .
Enzyme Inhibition Studies
The compound has been investigated for its role as an inhibitor of β-glucocerebrosidase, an enzyme linked to Gaucher disease. Studies have shown that certain derivatives based on this furanose structure can act as pharmacological chaperones, providing new avenues for therapeutic interventions .
Development of Functionalized Carbohydrates
In organic synthesis, this compound serves as a precursor for creating functionalized carbohydrate derivatives. These derivatives are crucial for studying carbohydrate-protein interactions and developing new biomaterials .
Case Study 1: Synthesis of Isoxazolidine Derivatives
A significant application of this compound was demonstrated in the synthesis of isoxazolidine derivatives through intramolecular cycloaddition reactions. Researchers reported high yields and stereoselectivity when using this compound as a substrate . The study highlighted how varying protecting groups can influence the reaction outcomes and product configurations.
Case Study 2: Antibacterial Activity Assessment
Another study evaluated the antibacterial activity of compounds derived from this compound against various bacterial strains, including methicillin-resistant Staphylococcus aureus (MRSA). The results indicated moderate to high antibacterial efficacy, suggesting potential applications in developing new antibiotics .
Table 1: Summary of Applications and Findings
Table 2: Antibacterial Activity Results
Compound | Bacterial Strain | MIC (µg/mL) |
---|---|---|
Isoxazolidine Derivative A | H. influenza ATCC 10211 | 0.25 - 0.5 |
Isoxazolidine Derivative B | E. faecalis SR7914 (vancomycin-resistant) | 4 - 8 |
Mecanismo De Acción
The mechanism of action of 2,3-O-Isopropylidene-D-erythrofuranose involves its role as an intermediate in various biochemical pathways. It interacts with specific molecular targets and pathways, facilitating the synthesis of biologically active compounds. The exact molecular targets and pathways involved depend on the specific application and the compounds being synthesized .
Comparación Con Compuestos Similares
Physical Properties :
- Appearance : Colorless syrup .
- Boiling Point : 112–113 °C at 25 mmHg .
- Solubility : Miscible in polar aprotic solvents (DCM, DMF, DMSO, MeOH, EtOAc) .
- Storage : Stable at 0 to -20 °C under inert conditions .
Comparison with Structurally Similar Compounds
Structural Variations in Isopropylidene-Protected Sugars
The position and number of isopropylidene groups significantly influence reactivity, solubility, and application. Below is a comparative analysis:
Table 1: Structural and Physical Comparison
Critical Analysis of Stability and Selectivity
- Steric Effects: The 2,3-O-isopropylidene group in erythrofuranose imposes steric hindrance, limiting reactivity at C-1 and C-4 but enhancing selectivity at C-5 .
- Solubility: Di-O-isopropylidene derivatives (e.g., glucofuranose) exhibit lower solubility in polar solvents due to increased hydrophobicity .
- Thermal Stability: Erythrofuranose derivatives decompose above 113 °C, whereas di-O-protected sugars (e.g., sorbofuranose) are stable up to 150 °C .
Actividad Biológica
Overview
2,3-O-Isopropylidene-D-erythrofuranose is a carbohydrate compound with the molecular formula CHO. This compound is characterized by a five-membered furanose ring structure and is primarily utilized as an intermediate in the synthesis of nucleoside analogues. Its unique stereochemistry and biological activity profile make it significant in various fields, particularly in medicinal chemistry and biochemistry.
Target of Action
The primary role of this compound is as a building block in the synthesis of nucleoside analogues. These analogues are crucial for inhibiting viral replication and cancer cell growth by mimicking natural nucleosides during nucleic acid synthesis.
Mode of Action
The compound integrates into nucleic acid chains during replication or transcription, potentially causing premature termination or introducing mutations. This action can inhibit critical enzymes such as reverse transcriptase and RNA polymerase, which are essential for viral replication and gene transcription .
This compound exhibits various biochemical properties that contribute to its functionality:
- Stability : The compound remains stable under ambient conditions but decomposes at elevated temperatures, releasing toxic gases.
- Reactivity : Its functional groups allow it to undergo several chemical transformations, making it versatile in synthetic organic chemistry.
Anticancer Properties
Recent studies have identified this compound as a synthetic anticancer agent. It inhibits protein synthesis by binding to sulfhydryl groups in cysteine residues within proteins. This mechanism suggests its potential application in cancer therapy .
Antiviral Activity
The compound's ability to act as a nucleoside analogue positions it as a candidate for antiviral drug development. By interfering with viral replication processes, it may contribute to therapeutic strategies against viral infections .
Research Applications
This compound is widely used in scientific research across multiple disciplines:
- Nucleoside Synthesis : As a precursor for synthesizing various nucleoside analogues that are vital in studying viral infections and cancer treatments.
- Carbohydrate Chemistry : Employed in spiroannulated carbohydrate synthesis and the development of sugar-based libraries for drug discovery .
Comparative Analysis with Similar Compounds
Compound Name | Structure Type | Unique Features |
---|---|---|
2,3-O-Isopropylidene-D-lyxofuranose | Furanose | Different stereochemistry at C-1 compared to erythrofuranose |
2,3-O-Isopropylidene-L-erythrofuranose | Furanose | Enantiomer with distinct biological activity profiles |
2,3-O-Isopropylidene-D-glucopyranose | Pyranose | Six-membered ring structure; different reactivity |
The comparison highlights the unique biological activity profile of this compound compared to its structural analogues. Its ability to inhibit protein synthesis sets it apart from other compounds that may not exhibit similar effects .
Case Studies and Research Findings
Several studies have explored the biological activities and applications of this compound:
- Anticancer Activity : A study demonstrated that this compound effectively inhibited the growth of specific cancer cell lines by disrupting protein synthesis pathways via cysteine modification .
- Nucleoside Analogue Development : Research focused on synthesizing novel nucleoside analogues from this compound has shown promising results in targeting viral infections and enhancing therapeutic efficacy against resistant strains .
Q & A
Basic Research Questions
Q. What are the established synthetic routes for 2,3-O-Isopropylidene-D-erythrofuranose, and how do reaction conditions vary across methodologies?
- Methodological Answer : The compound is synthesized via stereoselective protection of D-erythrose derivatives. A common approach involves reacting D-erythrofuranose with acetone under acid catalysis to form the isopropylidene protecting group. For example, Ho (1979) used formaldehyde to synthesize D-apiose derivatives, while Nachman et al. (1986) employed methyl 3-formyl intermediates to prepare branched-chain sugars . Variations in catalysts (e.g., H₂SO₄ vs. TsOH), solvent systems (e.g., anhydrous acetone vs. DMF), and temperature (room temperature vs. reflux) significantly impact yield and purity. Crystallization from ether/hexane mixtures is often used for purification .
Q. How is X-ray crystallography applied to confirm the structural configuration of this compound derivatives?
- Methodological Answer : Single-crystal X-ray diffraction (SCXRD) is critical for resolving stereochemistry. For instance, Robinson et al. (2009) determined the crystal structure of 2,3-O-Isopropylidene-3-C-phenylerythrofuranose, revealing a furanose ring puckering (C2′-endo) and isopropylidene group geometry (torsion angle ≈ 55°). Data collection at 173 K with Mo-Kα radiation (λ = 0.71073 Å) and refinement to R-factors <0.05 ensure accuracy. Hydrogen bonding networks and thermal displacement parameters further validate the structure .
Advanced Research Questions
Q. How can researchers address contradictions in NMR data when analyzing substituted derivatives of this compound?
- Methodological Answer : Discrepancies in proton coupling constants (e.g., J1',2') or carbon chemical shifts often arise from conformational flexibility or diastereomerism. Combining 2D NMR (COSY, HSQC) with computational modeling (DFT or molecular dynamics) helps assign signals. For example, Robinson et al. (2009) resolved ambiguities in cyclohexyl-substituted derivatives by correlating NOESY cross-peaks with SCXRD data to confirm axial vs. equatorial substituent orientation .
Q. What strategies improve stereoselectivity in Co(II)-mediated ring-opening reactions of 1,2-dioxane precursors to synthesize erythrono-γ-lactones?
- Methodological Answer : Co(II) complexes (e.g., Co(acac)₂) catalyze the stereoselective ring-opening of endoperoxides. Key factors include:
- Solvent polarity : Non-polar solvents (toluene) favor β-scission over epoxidation.
- Temperature : Low temperatures (–20°C) reduce side reactions.
- Ligand design : Bidentate ligands enhance regioselectivity. Pedersen et al. (2009) achieved >90% yield of erythrono-γ-lactones by optimizing these parameters, with stereochemistry confirmed via polarimetry and chiral HPLC .
Q. What experimental considerations are critical when comparing formaldehyde vs. other carbonyl sources for synthesizing D-apiose derivatives from this compound?
- Methodological Answer : Formaldehyde’s reactivity in aldol condensations must be balanced against competing side reactions (e.g., overalkylation). Ho (1979) used buffered conditions (pH 7–8) to control nucleophilicity, while Nachman et al. (1986) employed methyl formyl esters for milder activation. Kinetic studies (e.g., monitoring by <sup>13</sup>C NMR) and quantum mechanical calculations (e.g., transition state modeling) help identify rate-limiting steps and selectivity drivers .
Q. Data Contradiction and Optimization
Q. How should researchers interpret conflicting melting point data for this compound derivatives across literature sources?
- Methodological Answer : Discrepancies (e.g., mp 46–49°C vs. 52–54°C) often stem from polymorphism or impurities. Differential Scanning Calorimetry (DSC) can identify polymorphic transitions, while recrystallization in varying solvent systems (e.g., EtOH vs. EtOAc/hexane) isolates pure phases. For example, the di-O-isopropylidene derivative in showed mp variability depending on crystal packing, resolved via SCXRD and Hirshfeld surface analysis .
Q. Experimental Design
Q. What analytical techniques are essential for characterizing the regioselectivity of glycosylation reactions involving this compound?
- Methodological Answer : Regioselectivity is assessed via:
- Mass spectrometry (MS) : ESI-MS identifies molecular ions and fragmentation patterns.
- NMR spectroscopy : <sup>1</sup>H-<sup>13</sup>C HMBC correlations map glycosidic linkages.
- Enzymatic assays : Glycosidase digestion (e.g., α-mannosidase) confirms anomeric configuration.
For example, Robinson et al. (2006) combined these methods to validate the C3-phenyl derivative’s structure .
Propiedades
IUPAC Name |
(3aR,6aR)-2,2-dimethyl-3a,4,6,6a-tetrahydrofuro[3,4-d][1,3]dioxol-4-ol | |
---|---|---|
Source | PubChem | |
URL | https://pubchem.ncbi.nlm.nih.gov | |
Description | Data deposited in or computed by PubChem | |
InChI |
InChI=1S/C7H12O4/c1-7(2)10-4-3-9-6(8)5(4)11-7/h4-6,8H,3H2,1-2H3/t4-,5-,6?/m1/s1 | |
Source | PubChem | |
URL | https://pubchem.ncbi.nlm.nih.gov | |
Description | Data deposited in or computed by PubChem | |
InChI Key |
MBZCXOZTJSRAOQ-QYRBDRAASA-N | |
Source | PubChem | |
URL | https://pubchem.ncbi.nlm.nih.gov | |
Description | Data deposited in or computed by PubChem | |
Canonical SMILES |
CC1(OC2COC(C2O1)O)C | |
Source | PubChem | |
URL | https://pubchem.ncbi.nlm.nih.gov | |
Description | Data deposited in or computed by PubChem | |
Isomeric SMILES |
CC1(O[C@@H]2COC([C@@H]2O1)O)C | |
Source | PubChem | |
URL | https://pubchem.ncbi.nlm.nih.gov | |
Description | Data deposited in or computed by PubChem | |
Molecular Formula |
C7H12O4 | |
Source | PubChem | |
URL | https://pubchem.ncbi.nlm.nih.gov | |
Description | Data deposited in or computed by PubChem | |
Molecular Weight |
160.17 g/mol | |
Source | PubChem | |
URL | https://pubchem.ncbi.nlm.nih.gov | |
Description | Data deposited in or computed by PubChem | |
Retrosynthesis Analysis
AI-Powered Synthesis Planning: Our tool employs the Template_relevance Pistachio, Template_relevance Bkms_metabolic, Template_relevance Pistachio_ringbreaker, Template_relevance Reaxys, Template_relevance Reaxys_biocatalysis model, leveraging a vast database of chemical reactions to predict feasible synthetic routes.
One-Step Synthesis Focus: Specifically designed for one-step synthesis, it provides concise and direct routes for your target compounds, streamlining the synthesis process.
Accurate Predictions: Utilizing the extensive PISTACHIO, BKMS_METABOLIC, PISTACHIO_RINGBREAKER, REAXYS, REAXYS_BIOCATALYSIS database, our tool offers high-accuracy predictions, reflecting the latest in chemical research and data.
Strategy Settings
Precursor scoring | Relevance Heuristic |
---|---|
Min. plausibility | 0.01 |
Model | Template_relevance |
Template Set | Pistachio/Bkms_metabolic/Pistachio_ringbreaker/Reaxys/Reaxys_biocatalysis |
Top-N result to add to graph | 6 |
Feasible Synthetic Routes
Descargo de responsabilidad e información sobre productos de investigación in vitro
Tenga en cuenta que todos los artículos e información de productos presentados en BenchChem están destinados únicamente con fines informativos. Los productos disponibles para la compra en BenchChem están diseñados específicamente para estudios in vitro, que se realizan fuera de organismos vivos. Los estudios in vitro, derivados del término latino "in vidrio", involucran experimentos realizados en entornos de laboratorio controlados utilizando células o tejidos. Es importante tener en cuenta que estos productos no se clasifican como medicamentos y no han recibido la aprobación de la FDA para la prevención, tratamiento o cura de ninguna condición médica, dolencia o enfermedad. Debemos enfatizar que cualquier forma de introducción corporal de estos productos en humanos o animales está estrictamente prohibida por ley. Es esencial adherirse a estas pautas para garantizar el cumplimiento de los estándares legales y éticos en la investigación y experimentación.