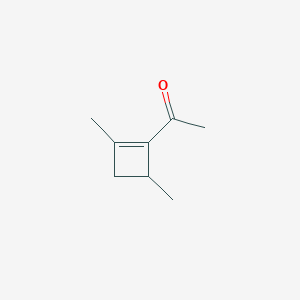
Ethanone, 1-(2,4-dimethyl-1-cyclobuten-1-YL)-(9CI)
- Haga clic en CONSULTA RÁPIDA para recibir una cotización de nuestro equipo de expertos.
- Con productos de calidad a un precio COMPETITIVO, puede centrarse más en su investigación.
Descripción general
Descripción
HDM-201, también conocido como siremadlin, es un potente e inhibitor selectivo de segunda generación de la proteína Murine Double Minute-2. Este compuesto es conocido por su capacidad para activar la vía p53, lo que lleva a efectos antiproliferativos en las células cancerosas. HDM-201 ha mostrado resultados prometedores en estudios preclínicos y clínicos, particularmente en el tratamiento de la leucemia mieloide aguda y otros cánceres con p53 de tipo salvaje .
Métodos De Preparación
Rutas sintéticas y condiciones de reacción
HDM-201 se sintetiza a través de un proceso de varios pasos que implica la preparación de compuestos intermedios seguidos de su acoplamiento y funcionalización. Los pasos clave incluyen:
Preparación de intermedios: La síntesis comienza con la preparación de intermedios clave a través de diversas reacciones orgánicas como la halogenación, la nitración y la reducción.
Reacciones de acoplamiento: Los intermedios se acoplan luego utilizando reacciones de acoplamiento cruzado catalizadas por paladio para formar la estructura central de HDM-201.
Métodos de producción industrial
La producción industrial de HDM-201 sigue rutas sintéticas similares, pero se optimiza para la fabricación a gran escala. Esto incluye el uso de reactores de alto rendimiento, química de flujo continuo y técnicas avanzadas de purificación para garantizar un alto rendimiento y pureza .
Análisis De Reacciones Químicas
Tipos de reacciones
HDM-201 experimenta diversas reacciones químicas, entre ellas:
Oxidación: HDM-201 puede oxidarse para formar intermedios reactivos que pueden reaccionar posteriormente con nucleófilos.
Reducción: El compuesto puede reducirse bajo condiciones específicas para producir diferentes análogos con diferentes actividades biológicas.
Sustitución: HDM-201 puede sufrir reacciones de sustitución donde los grupos funcionales se reemplazan por otros grupos para modificar sus propiedades.
Reactivos y condiciones comunes
Oxidación: Los agentes oxidantes comunes incluyen peróxido de hidrógeno y permanganato de potasio.
Reducción: Se utilizan agentes reductores como borohidruro de sodio e hidruro de aluminio y litio.
Sustitución: Se emplean varios nucleófilos y electrófilos en condiciones controladas para lograr las sustituciones deseadas.
Productos principales
Los principales productos formados a partir de estas reacciones incluyen análogos de HDM-201 con grupos funcionales modificados, que se estudian por sus actividades biológicas mejoradas o alteradas .
Aplicaciones Científicas De Investigación
HDM-201 tiene una amplia gama de aplicaciones de investigación científica, que incluyen:
Química: Se utiliza como compuesto herramienta para estudiar la vía p53 y su papel en la biología del cáncer.
Biología: Se emplea en ensayos basados en células para investigar los efectos de la activación de p53 en la regulación del ciclo celular y la apoptosis.
Medicina: Se encuentra en investigación clínica para el tratamiento de diversos cánceres, particularmente aquellos con p53 de tipo salvaje.
Industria: Aplicaciones potenciales en el desarrollo de nuevas terapias contra el cáncer y como compuesto líder para el descubrimiento de fármacos
Mecanismo De Acción
HDM-201 ejerce sus efectos inhibiendo la interacción entre la proteína p53 y la proteína Murine Double Minute-2. Esta inhibición estabiliza la proteína p53, lo que lleva a su activación. La p53 activada induce el arresto del ciclo celular y la apoptosis en las células cancerosas, ejerciendo así sus efectos antiproliferativos. Los objetivos moleculares y las vías involucradas incluyen la vía p53, que regula la expresión de genes involucrados en el control del ciclo celular y la apoptosis .
Comparación Con Compuestos Similares
HDM-201 se compara con otros compuestos similares como:
Nutlin-3: Otro inhibidor de Murine Double Minute-2, pero HDM-201 ha demostrado una mayor potencia y selectividad.
RG7388: Un inhibidor de Murine Double Minute-2 de segunda generación con mecanismos similares pero diferentes perfiles farmacocinéticos.
MI-77301: Otro potente inhibidor de Murine Double Minute-2 con propiedades químicas y actividades biológicas distintas.
HDM-201 es único debido a su alta selectividad para la interacción p53-Murine Double Minute-2 y sus perfiles farmacocinéticos y farmacodinámicos favorables .
Propiedades
IUPAC Name |
1-(2,4-dimethylcyclobuten-1-yl)ethanone |
Source
|
---|---|---|
Source | PubChem | |
URL | https://pubchem.ncbi.nlm.nih.gov | |
Description | Data deposited in or computed by PubChem | |
InChI |
InChI=1S/C8H12O/c1-5-4-6(2)8(5)7(3)9/h5H,4H2,1-3H3 |
Source
|
Source | PubChem | |
URL | https://pubchem.ncbi.nlm.nih.gov | |
Description | Data deposited in or computed by PubChem | |
InChI Key |
DBNPBZLNYQDORR-UHFFFAOYSA-N |
Source
|
Source | PubChem | |
URL | https://pubchem.ncbi.nlm.nih.gov | |
Description | Data deposited in or computed by PubChem | |
Canonical SMILES |
CC1CC(=C1C(=O)C)C |
Source
|
Source | PubChem | |
URL | https://pubchem.ncbi.nlm.nih.gov | |
Description | Data deposited in or computed by PubChem | |
Molecular Formula |
C8H12O |
Source
|
Source | PubChem | |
URL | https://pubchem.ncbi.nlm.nih.gov | |
Description | Data deposited in or computed by PubChem | |
Molecular Weight |
124.18 g/mol |
Source
|
Source | PubChem | |
URL | https://pubchem.ncbi.nlm.nih.gov | |
Description | Data deposited in or computed by PubChem | |
Descargo de responsabilidad e información sobre productos de investigación in vitro
Tenga en cuenta que todos los artículos e información de productos presentados en BenchChem están destinados únicamente con fines informativos. Los productos disponibles para la compra en BenchChem están diseñados específicamente para estudios in vitro, que se realizan fuera de organismos vivos. Los estudios in vitro, derivados del término latino "in vidrio", involucran experimentos realizados en entornos de laboratorio controlados utilizando células o tejidos. Es importante tener en cuenta que estos productos no se clasifican como medicamentos y no han recibido la aprobación de la FDA para la prevención, tratamiento o cura de ninguna condición médica, dolencia o enfermedad. Debemos enfatizar que cualquier forma de introducción corporal de estos productos en humanos o animales está estrictamente prohibida por ley. Es esencial adherirse a estas pautas para garantizar el cumplimiento de los estándares legales y éticos en la investigación y experimentación.