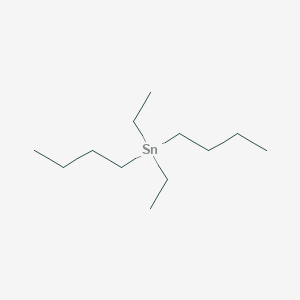
Estannano, dibutildietil-
Descripción general
Descripción
Stannane, dibutyldiethyl- is an organotin compound characterized by the presence of tin bonded to hydrocarbons. Organotin compounds, such as stannane, dibutyldiethyl-, are known for their applications in various fields due to their unique chemical properties. These compounds are part of the broader category of organometallic compounds, which contain tin-carbon bonds.
Aplicaciones Científicas De Investigación
Stannane, dibutyldiethyl- has several applications in scientific research:
Chemistry: Used as a reagent in organic synthesis for the formation of carbon-tin bonds.
Biology: Investigated for its potential use in biological systems due to its ability to interact with biomolecules.
Medicine: Explored for its potential use in drug delivery systems and as an antimicrobial agent.
Industry: Utilized in the production of polymers and as a stabilizer in the manufacturing of plastics.
Mecanismo De Acción
Target of Action
Stannanes are chemical compounds containing tin bonded to hydrocarbons . The primary targets of Stannane, dibutyldiethyl- are likely to be organic molecules that can undergo stannylation or C-Sn coupling reactions .
Mode of Action
The interaction of Stannane, dibutyldiethyl- with its targets involves the formation of C-Sn bonds . This process, known as stannylation, is a key step in the synthesis of various types of stannanes, including arylstannanes, alkylstannanes, vinylstannanes, and alkynylstannanes .
Biochemical Pathways
The compound’s role in the synthesis of various types of stannanes suggests that it may influence a range of biochemical reactions involving these compounds .
Pharmacokinetics
The compound’s molecular weight of 263008 may influence its bioavailability and pharmacokinetic profile.
Result of Action
The result of Stannane, dibutyldiethyl-'s action is the formation of various types of stannanes, which are versatile synthetic intermediates used in cross-coupling reactions and other applications in organic synthesis .
Action Environment
The action of Stannane, dibutyldiethyl- can be influenced by various environmental factors. For instance, the efficiency of stannylation reactions can be affected by the presence of other reactants, the reaction temperature, and the solvent used .
Métodos De Preparación
Synthetic Routes and Reaction Conditions: Stannane, dibutyldiethyl- can be synthesized through the reaction of diethylstannane with butyl lithium in an inert atmosphere. The reaction typically occurs in a solvent such as tetrahydrofuran at low temperatures to prevent decomposition. The reaction is as follows:
(C2H5)2SnH2+2BuLi→(C2H5)2Sn(Bu)2+2LiH
Industrial Production Methods: Industrial production of stannane, dibutyldiethyl- involves the use of large-scale reactors where the reaction conditions are carefully controlled to ensure high yield and purity. The process includes the purification of the product through distillation or recrystallization to remove any impurities.
Types of Reactions:
Oxidation: Stannane, dibutyldiethyl- can undergo oxidation reactions to form tin oxides. Common oxidizing agents include hydrogen peroxide and oxygen.
Reduction: Reduction reactions can convert stannane, dibutyldiethyl- to its corresponding hydrides using reducing agents such as lithium aluminum hydride.
Substitution: This compound can participate in substitution reactions where the butyl or ethyl groups are replaced by other functional groups. Common reagents include halogens and organolithium compounds.
Common Reagents and Conditions:
Oxidation: Hydrogen peroxide, oxygen; typically carried out at room temperature.
Reduction: Lithium aluminum hydride; reactions are conducted under anhydrous conditions.
Substitution: Halogens, organolithium compounds; reactions are performed in inert atmospheres to prevent unwanted side reactions.
Major Products Formed:
Oxidation: Tin oxides such as tin(IV) oxide.
Reduction: Tin hydrides.
Substitution: Various organotin compounds depending on the substituents used.
Comparación Con Compuestos Similares
- Stannane, dibutyldimethyl-
- Stannane, diethyldiethyl-
- Stannane, tributyl-
Comparison: Stannane, dibutyldiethyl- is unique due to its specific combination of butyl and ethyl groups, which confer distinct chemical properties compared to other organotin compounds. For example, stannane, dibutyldimethyl- has different steric and electronic properties due to the presence of methyl groups instead of ethyl groups. This affects its reactivity and applications in various fields.
Actividad Biológica
Stannane, dibutyldiethyl- (C10H24Sn), is an organotin compound that has garnered attention due to its biological activity and potential environmental impacts. This article explores the biological activity of dibutyldiethylstannane, focusing on its toxicity, interactions with biological systems, and implications for environmental health.
Dibutyldiethylstannane is characterized by its molecular formula C10H24Sn and a molecular weight of approximately 248.1 g/mol. It is a colorless to pale yellow liquid with a relatively low boiling point, which influences its behavior in environmental settings.
Property | Value |
---|---|
Molecular Weight | 248.1 g/mol |
Boiling Point | ~ 200 °C |
Solubility | Moderate in organic solvents |
Log Kow | 3.5 |
These properties suggest a moderate hydrophobicity, indicating potential bioaccumulation in aquatic organisms.
Toxicity Profile
Dibutyldiethylstannane exhibits significant toxicity towards various biological organisms, particularly aquatic species. Organotin compounds, including dibutyldiethylstannane, are known to disrupt endocrine functions and exhibit cytotoxic effects.
- Aquatic Toxicity : Studies have demonstrated that dibutyldiethylstannane can cause lethal and sub-lethal effects in fish and invertebrates. The mechanism of toxicity often involves interference with cellular signaling pathways and oxidative stress induction.
- Microbial Impact : Research indicates that dibutyldiethylstannane affects microbial communities in sediments, altering their composition and function. This can lead to reduced biodegradation capabilities of sediments, impacting overall ecosystem health .
The biological activity of dibutyldiethylstannane can be attributed to several mechanisms:
- Endocrine Disruption : Organotins can mimic or interfere with hormone signaling pathways, leading to reproductive and developmental issues in aquatic organisms.
- Oxidative Stress : The compound induces oxidative stress through the generation of reactive oxygen species (ROS), damaging cellular components such as DNA and lipids.
- Biodegradation Resistance : Certain microbial strains have shown resistance to dibutyldiethylstannane, indicating potential for bioremediation applications but also raising concerns about persistence in the environment .
Environmental Impact Studies
Several studies have focused on the environmental impacts of dibutyldiethylstannane:
- Sediment Microcosm Experiments : In controlled sediment microcosm studies, researchers observed that the presence of dibutyldiethylstannane significantly altered microbial community structures, favoring species capable of degrading organotin compounds while inhibiting others .
- Field Studies : Field studies in contaminated coastal environments have reported bioaccumulation of dibutyldiethylstannane in marine organisms, leading to trophic transfer and potential impacts on higher trophic levels.
Health Risk Assessments
Health risk assessments have been conducted to evaluate the potential human health risks associated with exposure to dibutyldiethylstannane through seafood consumption:
- Bioaccumulation Studies : Research indicates that marine organisms exposed to organotin compounds accumulate these toxins in their tissues, posing risks to human health through dietary intake .
- Regulatory Frameworks : Regulatory agencies are increasingly focusing on limiting the use of organotin compounds due to their persistent and toxic nature.
Propiedades
IUPAC Name |
dibutyl(diethyl)stannane | |
---|---|---|
Source | PubChem | |
URL | https://pubchem.ncbi.nlm.nih.gov | |
Description | Data deposited in or computed by PubChem | |
InChI |
InChI=1S/2C4H9.2C2H5.Sn/c2*1-3-4-2;2*1-2;/h2*1,3-4H2,2H3;2*1H2,2H3; | |
Source | PubChem | |
URL | https://pubchem.ncbi.nlm.nih.gov | |
Description | Data deposited in or computed by PubChem | |
InChI Key |
OLZKNGAFPKLTRN-UHFFFAOYSA-N | |
Source | PubChem | |
URL | https://pubchem.ncbi.nlm.nih.gov | |
Description | Data deposited in or computed by PubChem | |
Canonical SMILES |
CCCC[Sn](CC)(CC)CCCC | |
Source | PubChem | |
URL | https://pubchem.ncbi.nlm.nih.gov | |
Description | Data deposited in or computed by PubChem | |
Molecular Formula |
C12H28Sn | |
Source | PubChem | |
URL | https://pubchem.ncbi.nlm.nih.gov | |
Description | Data deposited in or computed by PubChem | |
DSSTOX Substance ID |
DTXSID70174520 | |
Record name | Stannane, dibutyldiethyl- | |
Source | EPA DSSTox | |
URL | https://comptox.epa.gov/dashboard/DTXSID70174520 | |
Description | DSSTox provides a high quality public chemistry resource for supporting improved predictive toxicology. | |
Molecular Weight |
291.06 g/mol | |
Source | PubChem | |
URL | https://pubchem.ncbi.nlm.nih.gov | |
Description | Data deposited in or computed by PubChem | |
CAS No. |
20525-62-6 | |
Record name | Stannane, dibutyldiethyl- | |
Source | ChemIDplus | |
URL | https://pubchem.ncbi.nlm.nih.gov/substance/?source=chemidplus&sourceid=0020525626 | |
Description | ChemIDplus is a free, web search system that provides access to the structure and nomenclature authority files used for the identification of chemical substances cited in National Library of Medicine (NLM) databases, including the TOXNET system. | |
Record name | Stannane, dibutyldiethyl- | |
Source | EPA DSSTox | |
URL | https://comptox.epa.gov/dashboard/DTXSID70174520 | |
Description | DSSTox provides a high quality public chemistry resource for supporting improved predictive toxicology. | |
Descargo de responsabilidad e información sobre productos de investigación in vitro
Tenga en cuenta que todos los artículos e información de productos presentados en BenchChem están destinados únicamente con fines informativos. Los productos disponibles para la compra en BenchChem están diseñados específicamente para estudios in vitro, que se realizan fuera de organismos vivos. Los estudios in vitro, derivados del término latino "in vidrio", involucran experimentos realizados en entornos de laboratorio controlados utilizando células o tejidos. Es importante tener en cuenta que estos productos no se clasifican como medicamentos y no han recibido la aprobación de la FDA para la prevención, tratamiento o cura de ninguna condición médica, dolencia o enfermedad. Debemos enfatizar que cualquier forma de introducción corporal de estos productos en humanos o animales está estrictamente prohibida por ley. Es esencial adherirse a estas pautas para garantizar el cumplimiento de los estándares legales y éticos en la investigación y experimentación.