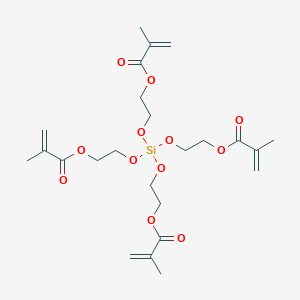
Tetrakis(2-methacryloxyethoxy)silane
- Haga clic en CONSULTA RÁPIDA para recibir una cotización de nuestro equipo de expertos.
- Con productos de calidad a un precio COMPETITIVO, puede centrarse más en su investigación.
Descripción general
Descripción
Tetrakis(2-methacryloxyethoxy)silane is a chemical compound that belongs to the class of silane coupling agents. It is commonly used in various scientific research applications due to its unique properties and versatile nature.
Mecanismo De Acción
Tetrakis(2-methacryloxyethoxy)silane works by forming covalent bonds between the silane coupling agent and the surface it is applied to. This results in the formation of a stable interface between two dissimilar materials. The methacrylate groups on the silane coupling agent can also undergo polymerization, resulting in the formation of a polymer layer on the surface.
Biochemical and Physiological Effects:
Tetrakis(2-methacryloxyethoxy)silane has not been extensively studied for its biochemical and physiological effects. However, it is considered to be non-toxic and has been used in various medical applications, including dental implants and bone tissue engineering.
Ventajas Y Limitaciones Para Experimentos De Laboratorio
Tetrakis(2-methacryloxyethoxy)silane has several advantages for lab experiments, including its ability to modify surfaces and enhance adhesion between dissimilar materials. It is also relatively easy to synthesize and has a long shelf life. However, it has some limitations, including its sensitivity to moisture and the need for specialized equipment for its application.
Direcciones Futuras
There are several future directions for the use of Tetrakis(2-methacryloxyethoxy)silane in scientific research. These include its use in the development of new materials with enhanced properties, such as self-healing materials and smart coatings. It can also be used in the development of new medical devices and drug delivery systems. Additionally, there is potential for its use in the field of nanotechnology, where it can be used to modify the surfaces of nanoparticles and enhance their properties.
In conclusion, Tetrakis(2-methacryloxyethoxy)silane is a versatile silane coupling agent that has numerous scientific research applications. Its ability to modify surfaces and enhance adhesion between dissimilar materials makes it a valuable tool in various fields, including polymer science, materials science, and nanotechnology. Its future directions include the development of new materials and medical devices, as well as its use in the field of nanotechnology.
Métodos De Síntesis
Tetrakis(2-methacryloxyethoxy)silane can be synthesized through the reaction of 3-mercaptopropyltrimethoxysilane with glycidyl methacrylate in the presence of a base catalyst. This reaction results in the formation of a silane coupling agent that has four methacrylate groups attached to a silicon atom.
Aplicaciones Científicas De Investigación
Tetrakis(2-methacryloxyethoxy)silane is widely used in scientific research due to its ability to modify surfaces and enhance the adhesion between dissimilar materials. It is commonly used in the fields of polymer science, materials science, and nanotechnology. Its applications include the modification of surfaces in dental implants, coatings for electronic devices, and adhesives for composite materials.
Propiedades
Número CAS |
116491-91-9 |
---|---|
Nombre del producto |
Tetrakis(2-methacryloxyethoxy)silane |
Fórmula molecular |
C24H36O12Si |
Peso molecular |
544.6 g/mol |
Nombre IUPAC |
2-[tris[2-(2-methylprop-2-enoyloxy)ethoxy]silyloxy]ethyl 2-methylprop-2-enoate |
InChI |
InChI=1S/C24H36O12Si/c1-17(2)21(25)29-9-13-33-37(34-14-10-30-22(26)18(3)4,35-15-11-31-23(27)19(5)6)36-16-12-32-24(28)20(7)8/h1,3,5,7,9-16H2,2,4,6,8H3 |
Clave InChI |
VICHSFFBPUZUOR-UHFFFAOYSA-N |
SMILES |
CC(=C)C(=O)OCCO[Si](OCCOC(=O)C(=C)C)(OCCOC(=O)C(=C)C)OCCOC(=O)C(=C)C |
SMILES canónico |
CC(=C)C(=O)OCCO[Si](OCCOC(=O)C(=C)C)(OCCOC(=O)C(=C)C)OCCOC(=O)C(=C)C |
Origen del producto |
United States |
Descargo de responsabilidad e información sobre productos de investigación in vitro
Tenga en cuenta que todos los artículos e información de productos presentados en BenchChem están destinados únicamente con fines informativos. Los productos disponibles para la compra en BenchChem están diseñados específicamente para estudios in vitro, que se realizan fuera de organismos vivos. Los estudios in vitro, derivados del término latino "in vidrio", involucran experimentos realizados en entornos de laboratorio controlados utilizando células o tejidos. Es importante tener en cuenta que estos productos no se clasifican como medicamentos y no han recibido la aprobación de la FDA para la prevención, tratamiento o cura de ninguna condición médica, dolencia o enfermedad. Debemos enfatizar que cualquier forma de introducción corporal de estos productos en humanos o animales está estrictamente prohibida por ley. Es esencial adherirse a estas pautas para garantizar el cumplimiento de los estándares legales y éticos en la investigación y experimentación.