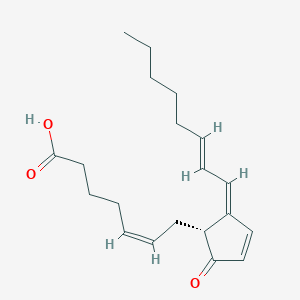
15d-Prostaglandin A2
Descripción general
Descripción
Synthesis Analysis
The synthesis of 15d-Prostaglandin A2 begins with the preparation of enantiopure 3-oxodicyclopentadiene by a lipase-mediated kinetic resolution. A three-component coupling followed by a retro-Diels−Alder reaction provides the C8 stereochemistry of the prostaglandin skeleton with high enantioselectivity .Molecular Structure Analysis
15d-Prostaglandin A2 has a unique and intricate molecular structure. It has a distinctive twenty-carbon skeleton, featuring a central five-membered ring. This ring contains oxygen atoms at specific positions, imparting reactivity and distinct chemical properties to prostaglandins .Chemical Reactions Analysis
15d-Prostaglandin A2 plays an important role in various chemical reactions. For instance, it has been found to enhance neuritogenesis and synaptogenesis in LA-N-5 human neuroblastoma cells .Physical And Chemical Properties Analysis
15d-Prostaglandin A2 has a molecular formula of C20H28O3, an average mass of 316.435 Da, and a monoisotopic mass of 316.203857 Da. It has 3 hydrogen bond acceptors, 1 hydrogen bond donor, and 11 freely rotating bonds .Aplicaciones Científicas De Investigación
Synthetic Analog of PGA2
“15-deoxy-delta12,14-Prostaglandin A2” is a synthetic analog of PGA2 . It shares common structural features with 15-deoxy-delta12,14-PGJ2, which is a ligand for PPARγ .
Ligand for PPARγ
This compound is a ligand for PPARγ . PPARγ (Peroxisome proliferator-activated receptor gamma) is a type of nuclear regulatory protein involved in the transcription of several genes. Ligands for PPARγ have potential applications in managing diseases like diabetes, obesity, and cardiovascular diseases .
Antimitotic and Antitumor Activity
Antimitotic and antitumor activity have been reported for a similar analog . This suggests that “15-deoxy-delta12,14-Prostaglandin A2” might also have potential applications in cancer treatment .
Effect on Lipid Accumulation
This compound has been used to study its effect on lipid accumulation . Understanding lipid accumulation can help in the study of diseases like atherosclerosis and obesity .
Viability/Mitochondrial Activity
“15-deoxy-delta12,14-Prostaglandin A2” has been used to study its effect on viability/mitochondrial activity . This could have implications in understanding cellular energy production and diseases related to mitochondrial dysfunction .
Role in Vascularization
The compound has been used to study the amount of vasculature in a vascularized adipose tissue model . This could be useful in understanding diseases related to blood vessels, such as atherosclerosis and hypertension .
Activation of Intestinal Fatty Acid Binding Protein (I-FABP)-PPARγ Pathway
It has been used as a peroxisome proliferator-activated receptor (PPARγ) agonist to activate the intestinal fatty acid binding protein (I-FABP)-PPARγ pathway . This pathway is important in lipid metabolism and could have implications in diseases like obesity and metabolic syndrome .
Induction of Apoptosis in Human Lung Cancer Cells
“15-deoxy-delta12,14-Prostaglandin A2” has been reported to inhibit human lung cancer cell growth through the induction of apoptosis . This suggests its potential application in lung cancer treatment .
Direcciones Futuras
Propiedades
IUPAC Name |
(Z)-7-[(1R,2Z)-2-[(E)-oct-2-enylidene]-5-oxocyclopent-3-en-1-yl]hept-5-enoic acid | |
---|---|---|
Source | PubChem | |
URL | https://pubchem.ncbi.nlm.nih.gov | |
Description | Data deposited in or computed by PubChem | |
InChI |
InChI=1S/C20H28O3/c1-2-3-4-5-6-9-12-17-15-16-19(21)18(17)13-10-7-8-11-14-20(22)23/h6-7,9-10,12,15-16,18H,2-5,8,11,13-14H2,1H3,(H,22,23)/b9-6+,10-7-,17-12-/t18-/m1/s1 | |
Source | PubChem | |
URL | https://pubchem.ncbi.nlm.nih.gov | |
Description | Data deposited in or computed by PubChem | |
InChI Key |
BHHHGDAJJMEHST-NBIYZLHXSA-N | |
Source | PubChem | |
URL | https://pubchem.ncbi.nlm.nih.gov | |
Description | Data deposited in or computed by PubChem | |
Canonical SMILES |
CCCCCC=CC=C1C=CC(=O)C1CC=CCCCC(=O)O | |
Source | PubChem | |
URL | https://pubchem.ncbi.nlm.nih.gov | |
Description | Data deposited in or computed by PubChem | |
Isomeric SMILES |
CCCCC/C=C/C=C\1/C=CC(=O)[C@@H]1C/C=C\CCCC(=O)O | |
Source | PubChem | |
URL | https://pubchem.ncbi.nlm.nih.gov | |
Description | Data deposited in or computed by PubChem | |
Molecular Formula |
C20H28O3 | |
Source | PubChem | |
URL | https://pubchem.ncbi.nlm.nih.gov | |
Description | Data deposited in or computed by PubChem | |
Molecular Weight |
316.4 g/mol | |
Source | PubChem | |
URL | https://pubchem.ncbi.nlm.nih.gov | |
Description | Data deposited in or computed by PubChem | |
Product Name |
15d-Prostaglandin A2 |
Retrosynthesis Analysis
AI-Powered Synthesis Planning: Our tool employs the Template_relevance Pistachio, Template_relevance Bkms_metabolic, Template_relevance Pistachio_ringbreaker, Template_relevance Reaxys, Template_relevance Reaxys_biocatalysis model, leveraging a vast database of chemical reactions to predict feasible synthetic routes.
One-Step Synthesis Focus: Specifically designed for one-step synthesis, it provides concise and direct routes for your target compounds, streamlining the synthesis process.
Accurate Predictions: Utilizing the extensive PISTACHIO, BKMS_METABOLIC, PISTACHIO_RINGBREAKER, REAXYS, REAXYS_BIOCATALYSIS database, our tool offers high-accuracy predictions, reflecting the latest in chemical research and data.
Strategy Settings
Precursor scoring | Relevance Heuristic |
---|---|
Min. plausibility | 0.01 |
Model | Template_relevance |
Template Set | Pistachio/Bkms_metabolic/Pistachio_ringbreaker/Reaxys/Reaxys_biocatalysis |
Top-N result to add to graph | 6 |
Feasible Synthetic Routes
Descargo de responsabilidad e información sobre productos de investigación in vitro
Tenga en cuenta que todos los artículos e información de productos presentados en BenchChem están destinados únicamente con fines informativos. Los productos disponibles para la compra en BenchChem están diseñados específicamente para estudios in vitro, que se realizan fuera de organismos vivos. Los estudios in vitro, derivados del término latino "in vidrio", involucran experimentos realizados en entornos de laboratorio controlados utilizando células o tejidos. Es importante tener en cuenta que estos productos no se clasifican como medicamentos y no han recibido la aprobación de la FDA para la prevención, tratamiento o cura de ninguna condición médica, dolencia o enfermedad. Debemos enfatizar que cualquier forma de introducción corporal de estos productos en humanos o animales está estrictamente prohibida por ley. Es esencial adherirse a estas pautas para garantizar el cumplimiento de los estándares legales y éticos en la investigación y experimentación.