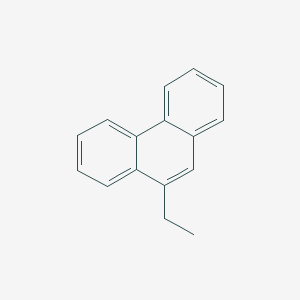
9-Ethylphenanthrene
Descripción general
Descripción
9-Ethylphenanthrene is a common component in air pollution and poses a severe potential hazard to human health . It is also suspected to have some estrogenic activity in rats .
Synthesis Analysis
The effect of alkyl substitution on the oxidative metabolism and mutagenicity of phenanthrene has been studied . The study investigated the effect of alkyl substitution on the CYP 450-mediated metabolism of phenanthrene and eight of its alkylated congeners .Molecular Structure Analysis
The molecular structure of 9-Ethylphenanthrene is represented by the formula C16H14 . The molecular weight is 206.2824 .Chemical Reactions Analysis
Alkyl substitution shifts the oxidative metabolism from the aromatic ring to the alkyl side chain . Increasing the length of the alkyl chain reduced overall metabolism . The position of the alkylation affects the metabolism and resulting mutagenicity of phenanthrene .Physical And Chemical Properties Analysis
9-Ethylphenanthrene is a solid substance . Its melting point ranges from 95 - 101 °C / 203 - 213.8 °F, and its boiling point is 336 °C / 636.8 °F .Aplicaciones Científicas De Investigación
Environmental Bioremediation
9-Ethylphenanthrene, as a polycyclic aromatic hydrocarbon (PAH), can be degraded by certain bacterial strains. Research has shown that Pseudomonas chlororaphis , an endophytic strain, can utilize phenanthrene and its derivatives for degradation . This process is crucial for the bioremediation of contaminated environments, such as soil and water bodies polluted by industrial activities.
Pharmaceuticals and Cosmetics Safety
Alkyl-substituted PAHs like 9-Ethylphenanthrene may end up in consumer products, raising safety concerns. Studies have investigated the oxidative metabolism and mutagenicity of alkyl-substituted PAHs, which is essential for assessing the safety of pharmaceuticals and cosmetics . Understanding the metabolic pathways can help in designing safer products and in regulatory assessments.
Food Safety
The unintentional consumption of PAHs through contaminated food is a significant safety issue. 9-Ethylphenanthrene’s presence in food, especially when derived from petrogenic sources, is an emerging health concern. Research on its bioactivation and metabolism contributes to the broader understanding of food safety and the potential risks associated with PAHs in the food supply .
Chemical Biology
The study of 9-Ethylphenanthrene’s interaction with biological systems falls under chemical biology. Its effects on cellular processes, gene expression, and metabolic pathways are of interest. This knowledge can lead to the development of new therapeutic agents or diagnostic tools that target specific pathways influenced by PAHs .
Organic Synthesis
9-Ethylphenanthrene can be used as a starting material or intermediate in organic synthesis. The regioselective functionalization of phenanthrenols, including 9-Ethylphenanthrene, is a field of study that explores methods to modify this compound at specific sites, which is valuable for creating complex organic molecules .
Mecanismo De Acción
Target of Action
It is known that polycyclic aromatic hydrocarbons (pahs), such as 9-ethylphenanthrene, can interact with various enzymes and proteins in the body .
Mode of Action
9-Ethylphenanthrene, like other PAHs, is believed to undergo bioactivation, a process that transforms the compound into a more reactive species . This bioactivation is mediated by enzymes such as the cytochrome P450 (CYP450) system . The bioactivated form of 9-Ethylphenanthrene can then interact with its targets, leading to various biological effects .
Biochemical Pathways
It is known that alkyl-substituted pahs like 9-ethylphenanthrene can shift the oxidative metabolism from the aromatic ring to the alkyl side chain . This shift in metabolism can lead to the formation of different metabolites and potentially affect various biochemical pathways .
Pharmacokinetics
It has been suggested that increasing the length of the alkyl chain can reduce the overall metabolism of alkyl-substituted pahs .
Result of Action
Some alkyl-substituted pahs have been shown to exhibit mutagenicity . For example, 1- and 9-methyl-phenanthrene, which have an additional bay region-like structural motif, showed mutagenicity toward Salmonella typhimurium TA98 and TA 100 .
Action Environment
The action of 9-Ethylphenanthrene can be influenced by various environmental factors. For instance, the presence of 9-Ethylphenanthrene in certain petroleum-derived products and in the environment can lead to its eventual uptake in consumer products, such as foodstuffs, cosmetics, and pharmaceuticals . This can raise safety concerns over possible exposure to alkylated PAHs .
Safety and Hazards
Propiedades
IUPAC Name |
9-ethylphenanthrene | |
---|---|---|
Source | PubChem | |
URL | https://pubchem.ncbi.nlm.nih.gov | |
Description | Data deposited in or computed by PubChem | |
InChI |
InChI=1S/C16H14/c1-2-12-11-13-7-3-4-9-15(13)16-10-6-5-8-14(12)16/h3-11H,2H2,1H3 | |
Source | PubChem | |
URL | https://pubchem.ncbi.nlm.nih.gov | |
Description | Data deposited in or computed by PubChem | |
InChI Key |
ZWANHXINCHPSIK-UHFFFAOYSA-N | |
Source | PubChem | |
URL | https://pubchem.ncbi.nlm.nih.gov | |
Description | Data deposited in or computed by PubChem | |
Canonical SMILES |
CCC1=CC2=CC=CC=C2C3=CC=CC=C31 | |
Source | PubChem | |
URL | https://pubchem.ncbi.nlm.nih.gov | |
Description | Data deposited in or computed by PubChem | |
Molecular Formula |
C16H14 | |
Source | PubChem | |
URL | https://pubchem.ncbi.nlm.nih.gov | |
Description | Data deposited in or computed by PubChem | |
DSSTOX Substance ID |
DTXSID90190202 | |
Record name | Phenanthrene, 9-ethyl- (8CI)(9CI) | |
Source | EPA DSSTox | |
URL | https://comptox.epa.gov/dashboard/DTXSID90190202 | |
Description | DSSTox provides a high quality public chemistry resource for supporting improved predictive toxicology. | |
Molecular Weight |
206.28 g/mol | |
Source | PubChem | |
URL | https://pubchem.ncbi.nlm.nih.gov | |
Description | Data deposited in or computed by PubChem | |
CAS RN |
3674-75-7 | |
Record name | 9-Ethylphenanthrene | |
Source | CAS Common Chemistry | |
URL | https://commonchemistry.cas.org/detail?cas_rn=3674-75-7 | |
Description | CAS Common Chemistry is an open community resource for accessing chemical information. Nearly 500,000 chemical substances from CAS REGISTRY cover areas of community interest, including common and frequently regulated chemicals, and those relevant to high school and undergraduate chemistry classes. This chemical information, curated by our expert scientists, is provided in alignment with our mission as a division of the American Chemical Society. | |
Explanation | The data from CAS Common Chemistry is provided under a CC-BY-NC 4.0 license, unless otherwise stated. | |
Record name | Phenanthrene, 9-ethyl- | |
Source | ChemIDplus | |
URL | https://pubchem.ncbi.nlm.nih.gov/substance/?source=chemidplus&sourceid=0003674757 | |
Description | ChemIDplus is a free, web search system that provides access to the structure and nomenclature authority files used for the identification of chemical substances cited in National Library of Medicine (NLM) databases, including the TOXNET system. | |
Record name | 9-ETHYLPHENANTHRENE | |
Source | DTP/NCI | |
URL | https://dtp.cancer.gov/dtpstandard/servlet/dwindex?searchtype=NSC&outputformat=html&searchlist=226072 | |
Description | The NCI Development Therapeutics Program (DTP) provides services and resources to the academic and private-sector research communities worldwide to facilitate the discovery and development of new cancer therapeutic agents. | |
Explanation | Unless otherwise indicated, all text within NCI products is free of copyright and may be reused without our permission. Credit the National Cancer Institute as the source. | |
Record name | Phenanthrene, 9-ethyl- (8CI)(9CI) | |
Source | EPA DSSTox | |
URL | https://comptox.epa.gov/dashboard/DTXSID90190202 | |
Description | DSSTox provides a high quality public chemistry resource for supporting improved predictive toxicology. | |
Record name | 9-Ethylphenanthrene | |
Source | FDA Global Substance Registration System (GSRS) | |
URL | https://gsrs.ncats.nih.gov/ginas/app/beta/substances/4T3XJL8RBB | |
Description | The FDA Global Substance Registration System (GSRS) enables the efficient and accurate exchange of information on what substances are in regulated products. Instead of relying on names, which vary across regulatory domains, countries, and regions, the GSRS knowledge base makes it possible for substances to be defined by standardized, scientific descriptions. | |
Explanation | Unless otherwise noted, the contents of the FDA website (www.fda.gov), both text and graphics, are not copyrighted. They are in the public domain and may be republished, reprinted and otherwise used freely by anyone without the need to obtain permission from FDA. Credit to the U.S. Food and Drug Administration as the source is appreciated but not required. | |
Retrosynthesis Analysis
AI-Powered Synthesis Planning: Our tool employs the Template_relevance Pistachio, Template_relevance Bkms_metabolic, Template_relevance Pistachio_ringbreaker, Template_relevance Reaxys, Template_relevance Reaxys_biocatalysis model, leveraging a vast database of chemical reactions to predict feasible synthetic routes.
One-Step Synthesis Focus: Specifically designed for one-step synthesis, it provides concise and direct routes for your target compounds, streamlining the synthesis process.
Accurate Predictions: Utilizing the extensive PISTACHIO, BKMS_METABOLIC, PISTACHIO_RINGBREAKER, REAXYS, REAXYS_BIOCATALYSIS database, our tool offers high-accuracy predictions, reflecting the latest in chemical research and data.
Strategy Settings
Precursor scoring | Relevance Heuristic |
---|---|
Min. plausibility | 0.01 |
Model | Template_relevance |
Template Set | Pistachio/Bkms_metabolic/Pistachio_ringbreaker/Reaxys/Reaxys_biocatalysis |
Top-N result to add to graph | 6 |
Feasible Synthetic Routes
Q & A
Q1: What is the significance of 9-Ethylphenanthrene in environmental science?
A1: 9-Ethylphenanthrene, alongside other alkylated phenanthrenes, is a significant component of crude oil. The Deepwater Horizon oil spill released substantial amounts of these compounds into the environment. [] Understanding the behavior and effects of 9-Ethylphenanthrene is crucial for assessing the environmental and health risks associated with such oil spills.
Q2: How does 9-Ethylphenanthrene interact with living organisms?
A2: Research on human hepatoma (HepG2) cells reveals that 9-Ethylphenanthrene undergoes metabolic activation through various pathways, including side-chain hydroxylation and the diol-epoxide pathway. [] This activation leads to the formation of metabolites like tetraols and O-monosulfonated-catechols. [] These metabolites could potentially serve as biomarkers for human exposure to 9-Ethylphenanthrene. [] Studies on sheepshead minnows (Cyprinodon variegatus) show that 9-Ethylphenanthrene is bioconcentrated and biotransformed in these fish. [] The rate of uptake and elimination is influenced by the degree of alkylation. []
Q3: How does the structure of 9-Ethylphenanthrene relate to its properties?
A3: The presence of the ethyl group in 9-Ethylphenanthrene influences its binding affinity to humic acid, impacting its environmental fate and bioavailability. [, ] Research indicates that the binding affinity of 9-Ethylphenanthrene to humic acid is higher than that of phenanthrene but similar to retene. [, ] This difference is attributed to the alkyl group's influence on hydrophobicity and its ability to fit within the hydrophobic cavities of humic acid. [, ] Additionally, the ethyl group affects the fluorescence properties compared to unsubstituted phenanthrene. []
Q4: Are there analytical methods for detecting 9-Ethylphenanthrene?
A4: Yes, synchronous fluorimetry, using hydroxyl-propyl beta-cyclodextrin as a sensitizer, can simultaneously determine 9-Ethylphenanthrene, pyrene, and 1-hydroxypyrene in aqueous solutions. [] Additionally, laser-induced nanosecond time-resolved fluorescence (LITRF) is a novel approach for investigating the interactions between 9-Ethylphenanthrene and humic acid. [, ]
Q5: What historical research exists on 9-Ethylphenanthrene?
A5: Early research in the 1930s explored the estrogenic activity of various synthetic phenanthrene compounds, including 9-Ethylphenanthrene. [] While 9-Ethylphenanthrene showed some estrogenic activity at high doses, it was significantly less potent than natural estrogens like theelol. [] This research highlights the diverse biological activities that can be associated with polycyclic aromatic hydrocarbons and their derivatives.
Descargo de responsabilidad e información sobre productos de investigación in vitro
Tenga en cuenta que todos los artículos e información de productos presentados en BenchChem están destinados únicamente con fines informativos. Los productos disponibles para la compra en BenchChem están diseñados específicamente para estudios in vitro, que se realizan fuera de organismos vivos. Los estudios in vitro, derivados del término latino "in vidrio", involucran experimentos realizados en entornos de laboratorio controlados utilizando células o tejidos. Es importante tener en cuenta que estos productos no se clasifican como medicamentos y no han recibido la aprobación de la FDA para la prevención, tratamiento o cura de ninguna condición médica, dolencia o enfermedad. Debemos enfatizar que cualquier forma de introducción corporal de estos productos en humanos o animales está estrictamente prohibida por ley. Es esencial adherirse a estas pautas para garantizar el cumplimiento de los estándares legales y éticos en la investigación y experimentación.