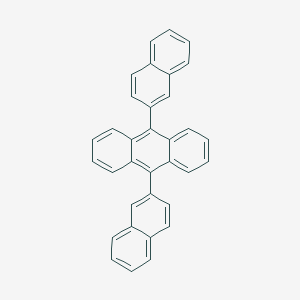
9,10-Di(naphthalen-2-yl)anthracene
Descripción general
Descripción
9,10-Di(naphthalen-2-yl)anthracene is an organic compound that belongs to the family of anthracene derivatives. It is known for its high thermal stability and morphological stability, making it a promising material for various applications, particularly in the field of organic electronics . This compound is widely used as a host material in blue organic light-emitting diodes (OLEDs) due to its wide energy band gap and excellent luminescent properties .
Aplicaciones Científicas De Investigación
9,10-Di(naphthalen-2-yl)anthracene has a wide range of scientific research applications, including:
Biology: The compound’s luminescent properties make it useful in bioimaging and as a fluorescent probe for detecting biological molecules.
Medicine: Research is ongoing to explore its potential in photodynamic therapy and other medical applications.
Mecanismo De Acción
Target of Action
The primary target of 9,10-Di(naphthalen-2-yl)anthracene (also known as ADN) is the organic light-emitting diode (OLED) devices . It is used as a host material in the emissive layer of OLEDs .
Mode of Action
ADN interacts with its targets by emitting light. It is luminescent, emitting both fluorescent and phosphorescent light . The compound is fabricated using the Suzuki coupling reaction .
Biochemical Pathways
ADN affects the electroluminescence (EL) performances of OLED devices . It is used as a blue fluorescent emissive material . The UV-Vis absorption, fluorescence emission spectroscopy, and cyclic voltammetry (CV) are carried out to investigate the relationships between the physical properties and molecular structures .
Result of Action
The result of ADN’s action is the emission of light. Specifically, it is used to achieve highly efficient sky-blue emission in OLED devices . For instance, a device based on OCADN, a derivative of ADN, exhibited highly efficient sky-blue emission with a current efficiency of 2.25 cd A −1, power efficiency of 1.13 lm W −1 and CIE ( x, y) coordinate of (0.16, 0.30) at 8 V .
Análisis Bioquímico
Biochemical Properties
It is known that this compound and its derivatives have been used as blue fluorescent emissive materials
Cellular Effects
Given its use in OLEDs, it may influence cellular function through its light-emitting properties .
Molecular Mechanism
It is known to exhibit blue fluorescence when used in OLEDs
Temporal Effects in Laboratory Settings
Métodos De Preparación
Synthetic Routes and Reaction Conditions
The synthesis of 9,10-Di(naphthalen-2-yl)anthracene typically involves the use of aromatic nucleophilic substitution reactions. One common method is to start with anthracene and introduce naphthyl groups at the 9 and 10 positions through chemical reactions under specific conditions . The Suzuki reaction, Wittig reaction, and Heck reaction are commonly employed to synthesize this compound .
Industrial Production Methods
In industrial settings, the production of this compound may involve large-scale chemical reactions using similar synthetic routes. The reactions are optimized for higher yields and purity, ensuring the compound meets the required standards for its applications in OLEDs and other electronic devices.
Análisis De Reacciones Químicas
Types of Reactions
9,10-Di(naphthalen-2-yl)anthracene undergoes various chemical reactions, including:
Oxidation: This reaction can lead to the formation of quinones or other oxidized derivatives.
Reduction: Reduction reactions can convert the compound into its reduced forms, potentially altering its electronic properties.
Substitution: Aromatic substitution reactions can introduce different functional groups to the anthracene core, modifying its properties.
Common Reagents and Conditions
Oxidation: Common oxidizing agents include potassium permanganate (KMnO₄) and chromium trioxide (CrO₃).
Reduction: Reducing agents such as lithium aluminum hydride (LiAlH₄) and sodium borohydride (NaBH₄) are often used.
Major Products
The major products formed from these reactions depend on the specific reagents and conditions used. For example, oxidation can yield quinones, while substitution reactions can produce various functionalized anthracene derivatives .
Comparación Con Compuestos Similares
Similar Compounds
9,10-Diphenylanthracene: Another anthracene derivative used in OLEDs.
2-Methyl-9,10-di(naphthalen-2-yl)anthracene: A similar compound with a methyl group at the 2-position.
9,10-Di(2-naphthyl)anthracene: A closely related compound with similar applications.
Uniqueness
9,10-Di(naphthalen-2-yl)anthracene stands out due to its high thermal stability, wide energy band gap, and excellent luminescent properties. These characteristics make it a superior choice for use in blue OLEDs and other advanced electronic applications .
Propiedades
IUPAC Name |
9,10-dinaphthalen-2-ylanthracene | |
---|---|---|
Source | PubChem | |
URL | https://pubchem.ncbi.nlm.nih.gov | |
Description | Data deposited in or computed by PubChem | |
InChI |
InChI=1S/C34H22/c1-3-11-25-21-27(19-17-23(25)9-1)33-29-13-5-7-15-31(29)34(32-16-8-6-14-30(32)33)28-20-18-24-10-2-4-12-26(24)22-28/h1-22H | |
Source | PubChem | |
URL | https://pubchem.ncbi.nlm.nih.gov | |
Description | Data deposited in or computed by PubChem | |
InChI Key |
VIZUPBYFLORCRA-UHFFFAOYSA-N | |
Source | PubChem | |
URL | https://pubchem.ncbi.nlm.nih.gov | |
Description | Data deposited in or computed by PubChem | |
Canonical SMILES |
C1=CC=C2C=C(C=CC2=C1)C3=C4C=CC=CC4=C(C5=CC=CC=C53)C6=CC7=CC=CC=C7C=C6 | |
Source | PubChem | |
URL | https://pubchem.ncbi.nlm.nih.gov | |
Description | Data deposited in or computed by PubChem | |
Molecular Formula |
C34H22 | |
Source | PubChem | |
URL | https://pubchem.ncbi.nlm.nih.gov | |
Description | Data deposited in or computed by PubChem | |
DSSTOX Substance ID |
DTXSID10619329 | |
Record name | 9,10-Di(naphthalen-2-yl)anthracene | |
Source | EPA DSSTox | |
URL | https://comptox.epa.gov/dashboard/DTXSID10619329 | |
Description | DSSTox provides a high quality public chemistry resource for supporting improved predictive toxicology. | |
Molecular Weight |
430.5 g/mol | |
Source | PubChem | |
URL | https://pubchem.ncbi.nlm.nih.gov | |
Description | Data deposited in or computed by PubChem | |
CAS No. |
122648-99-1 | |
Record name | 9,10-Di(naphthalen-2-yl)anthracene | |
Source | EPA DSSTox | |
URL | https://comptox.epa.gov/dashboard/DTXSID10619329 | |
Description | DSSTox provides a high quality public chemistry resource for supporting improved predictive toxicology. | |
Record name | 9,10-Di(2-naphthyl)anthracene | |
Source | European Chemicals Agency (ECHA) | |
URL | https://echa.europa.eu/information-on-chemicals | |
Description | The European Chemicals Agency (ECHA) is an agency of the European Union which is the driving force among regulatory authorities in implementing the EU's groundbreaking chemicals legislation for the benefit of human health and the environment as well as for innovation and competitiveness. | |
Explanation | Use of the information, documents and data from the ECHA website is subject to the terms and conditions of this Legal Notice, and subject to other binding limitations provided for under applicable law, the information, documents and data made available on the ECHA website may be reproduced, distributed and/or used, totally or in part, for non-commercial purposes provided that ECHA is acknowledged as the source: "Source: European Chemicals Agency, http://echa.europa.eu/". Such acknowledgement must be included in each copy of the material. ECHA permits and encourages organisations and individuals to create links to the ECHA website under the following cumulative conditions: Links can only be made to webpages that provide a link to the Legal Notice page. | |
Retrosynthesis Analysis
AI-Powered Synthesis Planning: Our tool employs the Template_relevance Pistachio, Template_relevance Bkms_metabolic, Template_relevance Pistachio_ringbreaker, Template_relevance Reaxys, Template_relevance Reaxys_biocatalysis model, leveraging a vast database of chemical reactions to predict feasible synthetic routes.
One-Step Synthesis Focus: Specifically designed for one-step synthesis, it provides concise and direct routes for your target compounds, streamlining the synthesis process.
Accurate Predictions: Utilizing the extensive PISTACHIO, BKMS_METABOLIC, PISTACHIO_RINGBREAKER, REAXYS, REAXYS_BIOCATALYSIS database, our tool offers high-accuracy predictions, reflecting the latest in chemical research and data.
Strategy Settings
Precursor scoring | Relevance Heuristic |
---|---|
Min. plausibility | 0.01 |
Model | Template_relevance |
Template Set | Pistachio/Bkms_metabolic/Pistachio_ringbreaker/Reaxys/Reaxys_biocatalysis |
Top-N result to add to graph | 6 |
Feasible Synthetic Routes
Q1: What makes ADN derivatives suitable for use in organic light-emitting diodes (OLEDs)?
A1: ADN derivatives are promising blue fluorescent emissive materials for OLEDs due to their favorable optical properties. Research has shown that modifying the ADN core structure by introducing various aromatic groups at specific positions can fine-tune the emission color and enhance device efficiency . For instance, a device utilizing an ADN derivative with an octyl chain substitution (OCADN) exhibited efficient sky-blue emission with a current efficiency of 2.25 cd A-1 .
Q2: How does the alkyl group at the 2-position of ADN affect its performance in solution-processed OLEDs?
A2: The presence of alkyl groups, such as methyl or tert-butyl, at the 2-position of ADN significantly influences its film-forming properties in solution processing. Studies comparing ADN, 2-Methyl-9,10-di-naphthalen-2-yl-anthracene (MADN), and 2-tert-butyl-9,10-di-naphthalen-2-yl-anthracene (TBADN) revealed that while the alkylated derivatives formed amorphous films suitable for light emission, unsubstituted ADN exhibited crystallinity, leading to increased leakage current and diminished device performance .
Q3: Can ADN be formulated into nanoparticles for biological applications?
A3: Yes, a study demonstrated the fabrication of water-dispersible and highly luminescent nanoparticles using the ADN derivative TBADN . These nanoparticles, surface-modified with an amphiphilic polymer, exhibited high dye-loading capacity and impressive brightness, surpassing that of some quantum dots. Furthermore, the researchers successfully conjugated folic acid to these nanoparticles, highlighting their potential for targeted cell imaging applications .
Q4: How does the choice of alkali metal alkylcarboxylate impact the performance of ADN-based OLEDs?
A4: The choice of alkali metal alkylcarboxylate as an electron injection material in ADN-based OLEDs significantly affects device performance. Studies have shown that while devices with cesium salts like (CH3)3CCOOCs maintain consistent performance regardless of the alkyl chain length, using rubidium or cesium salts becomes crucial for efficient electron injection when incorporating certain electron transport layers like 2-methyl-9,10-di(naphthalen-2-yl)anthracene (m-ADN) .
Q5: Are there any environmental concerns associated with ADN and its derivatives?
A5: While the provided research doesn't specifically address the environmental impact of ADN and its derivatives, it is crucial to consider potential ecotoxicological effects and explore strategies for responsible waste management and recycling. Further research is needed to assess the long-term environmental consequences and develop sustainable practices for the synthesis, application, and disposal of these compounds.
Descargo de responsabilidad e información sobre productos de investigación in vitro
Tenga en cuenta que todos los artículos e información de productos presentados en BenchChem están destinados únicamente con fines informativos. Los productos disponibles para la compra en BenchChem están diseñados específicamente para estudios in vitro, que se realizan fuera de organismos vivos. Los estudios in vitro, derivados del término latino "in vidrio", involucran experimentos realizados en entornos de laboratorio controlados utilizando células o tejidos. Es importante tener en cuenta que estos productos no se clasifican como medicamentos y no han recibido la aprobación de la FDA para la prevención, tratamiento o cura de ninguna condición médica, dolencia o enfermedad. Debemos enfatizar que cualquier forma de introducción corporal de estos productos en humanos o animales está estrictamente prohibida por ley. Es esencial adherirse a estas pautas para garantizar el cumplimiento de los estándares legales y éticos en la investigación y experimentación.