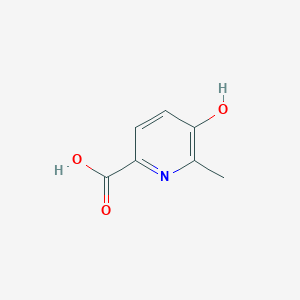
5-Hydroxy-6-methylpyridine-2-carboxylic acid
Descripción general
Descripción
5-Hydroxy-6-methylpyridine-2-carboxylic acid (CAS: 121447-41-4) is a pyridine derivative with the molecular formula C₇H₇NO₃ and a molecular weight of 153.14 g/mol . Its structure features a hydroxyl (-OH) group at position 5, a methyl (-CH₃) group at position 6, and a carboxylic acid (-COOH) group at position 2 (Figure 1). This compound exhibits a polar surface area (PSA) of 70.42 Ų and a LogP value of 0.79, indicating moderate hydrophilicity .
Mecanismo De Acción
Target of Action
Mode of Action
5-Hydroxy-6-methylpyridine-2-carboxylic acid interacts with its targets through chemical reactions. It reacts with carboxylic acids RCOOH to give an equilibrium mixture of products. It also plays a role in the oxidation of 5-formyl-3-hydroxy-2-methylpyridine 4-carboxylic acid to 3-hydroxy-2-methylpyridine 4,5-dicarboxylic acid with NAD+.
Biochemical Pathways
Análisis Bioquímico
Biochemical Properties
It has been used as a starting reagent for the synthesis of other compounds . It has also been used as a ligand of a platinum complex, which showed protein kinase inhibitory action at nanomolar levels . This suggests that 5-Hydroxy-6-methylpyridine-2-carboxylic acid may interact with enzymes and proteins in biochemical reactions.
Cellular Effects
Given its role as a ligand in a platinum complex with protein kinase inhibitory action , it may influence cell function by modulating protein kinase activity. Protein kinases play crucial roles in cell signaling pathways, gene expression, and cellular metabolism.
Molecular Mechanism
As a ligand in a platinum complex, it may exert its effects at the molecular level through binding interactions with biomolecules, potentially leading to enzyme inhibition or activation and changes in gene expression .
Actividad Biológica
Introduction
5-Hydroxy-6-methylpyridine-2-carboxylic acid (also known as 5-Hydroxy-6-methylpyridine-2-carboxylate) is a pyridine derivative with notable biological activities. This compound has garnered attention in various fields, including medicinal chemistry and biochemistry, due to its potential therapeutic applications. This article reviews the biological activity of this compound, focusing on its mechanisms of action, pharmacological effects, and relevant case studies.
This compound has the following chemical properties:
- Molecular Formula : C7H7NO3
- Molecular Weight : 153.14 g/mol
- CAS Number : 121447-41-4
- Structural Formula :
Enzymatic Interactions
Research indicates that this compound may act as a substrate or inhibitor for various enzymes. For example, studies have shown that it can interact with enzymes involved in metabolic pathways, potentially modulating their activity.
Antioxidant Activity
Several studies have highlighted the antioxidant properties of this compound. It is believed to scavenge free radicals, thereby reducing oxidative stress in cells. This activity is crucial for protecting cellular components from damage, which is particularly relevant in neurodegenerative diseases.
Neuroprotective Effects
The compound has demonstrated neuroprotective effects in various in vitro studies. It appears to enhance neuronal survival under stress conditions, possibly through the modulation of signaling pathways related to apoptosis and inflammation.
Antimicrobial Properties
This compound exhibits antimicrobial activity against several bacterial strains. In a study involving Burkholderia sp. MAK1, the compound was shown to enhance the conversion of pyridine derivatives into hydroxylated products, indicating its role as a biocatalyst in microbial metabolism .
Cytotoxicity Studies
In cytotoxicity assays, this compound has been tested against various cancer cell lines. Results indicated that it can induce apoptosis in certain cancer cells, suggesting potential for development as an anticancer agent.
Pharmacokinetics
The pharmacokinetic profile of this compound includes good solubility and absorption characteristics. Studies suggest it is metabolized primarily in the liver and excreted via renal pathways.
Case Study 1: Neuroprotection in Animal Models
A study investigated the neuroprotective effects of this compound in rodent models of neurodegeneration. The results showed significant improvement in cognitive function and reduced markers of oxidative stress following treatment with this compound.
Case Study 2: Antimicrobial Efficacy
In a clinical trial assessing the antimicrobial efficacy of this compound against Staphylococcus aureus, the compound exhibited significant inhibition of bacterial growth at low concentrations, suggesting its potential as a therapeutic agent for bacterial infections.
Study | Findings | |
---|---|---|
Neuroprotection | Improved cognitive function in rodents | Potential treatment for neurodegenerative diseases |
Antimicrobial | Significant growth inhibition of Staphylococcus aureus | Potential therapeutic agent for bacterial infections |
Aplicaciones Científicas De Investigación
Biological Activities
Antimicrobial Properties
Research indicates that 5-HMPCA exhibits significant antibacterial and antifungal activities. Studies have demonstrated its effectiveness against various pathogens, suggesting its potential as a lead compound for developing new antimicrobial agents. For instance, laboratory tests have shown that 5-HMPCA can inhibit the growth of specific bacterial strains, making it a candidate for further pharmacological development.
Enzyme Modulation
The compound's structural features allow it to modulate enzyme activity. It can act as both a substrate and an inhibitor in enzymatic reactions. This dual functionality highlights its potential in drug design, particularly in targeting enzymes involved in metabolic pathways associated with diseases.
Applications in Catalysis
5-HMPCA has been explored for its catalytic properties due to its ability to interact with metal ions. Studies suggest that it can serve as a ligand in various catalytic processes, particularly in organic synthesis. Its interactions with transition metals may facilitate reactions such as oxidation and reduction, making it useful in synthetic organic chemistry.
Material Science
The compound's unique properties have led to its application in material science, particularly in the development of biosensors and biofuel cells. For example, research has indicated that 5-HMPCA can be incorporated into carbon nanotube matrices to enhance the performance of glucose biosensors. This application leverages its ability to facilitate electron transfer between enzymes and electrodes .
Case Studies
-
Antimicrobial Efficacy Study
A study conducted on various bacterial strains demonstrated that 5-HMPCA inhibited bacterial growth effectively at concentrations as low as 100 µg/mL. The results indicated that the compound could serve as a potential template for developing new antibiotics targeting resistant bacterial strains. -
Enzymatic Activity Modulation
In vitro assays showed that 5-HMPCA could inhibit specific enzymes involved in metabolic pathways linked to cancer cell proliferation. This finding suggests that further investigation into its role as an anticancer agent could be warranted. -
Biosensor Development
Research involving glucose biosensors revealed that integrating 5-HMPCA into carbon nanotube matrices significantly improved sensor sensitivity and response time. The study highlighted its potential application in developing efficient biosensing devices for glucose monitoring in diabetic patients .
Q & A
Basic Research Questions
Q. What synthetic methodologies are reported for 5-hydroxy-6-methylpyridine-2-carboxylic acid, and how can reaction conditions be systematically optimized?
The synthesis of pyridinecarboxylic acid derivatives often involves oxidation of methyl-substituted precursors. For example, 5-methoxypyridine-2-carboxylic acid was synthesized by oxidizing 5-methoxy-2-methylpyridine with potassium permanganate (KMnO₄) under reflux (90–95°C) in water, yielding 47% after copper salt precipitation and pH-controlled crystallization . Key optimization parameters include:
- Oxidant stoichiometry : Excess KMnO₄ (0.44 mol per 0.1 mol substrate) ensures complete oxidation of methyl groups.
- Temperature control : Maintaining 90–95°C minimizes side reactions.
- Workup strategy : Adjusting the pH to 4 during crystallization enhances purity. For 6-substituted analogs, nucleophilic substitution (e.g., NaOMe/MeOH reflux) followed by acidification improved yields to 84%, highlighting the importance of regioselective protection .
Q. How can spectroscopic and analytical techniques (e.g., NMR, elemental analysis) validate the structure and purity of this compound?
Structural validation relies on:
- ¹H NMR : Pyridine proton signals (e.g., δ 7.4–8.3 ppm for aromatic protons) and hydroxyl/COOH resonances (broad peaks at δ 9.2–9.8 ppm) confirm substitution patterns .
- Elemental analysis : Matching calculated vs. experimental values for C, H, and N (e.g., C: 54.92% calc. vs. 54.61% found) ensures purity .
- Melting point consistency : Sharp melting points (e.g., 167°C for 5-methoxy analogs) indicate homogeneity .
Advanced Research Questions
Q. What factors contribute to yield discrepancies in the synthesis of pyridinecarboxylic acids, and how can competing reaction pathways be mitigated?
Yield variations arise from:
- Regioselectivity challenges : Competing oxidation at alternate positions (e.g., 5- vs. 6-substitution) can reduce efficiency. For 6-methoxy analogs, methoxy groups may sterically hinder oxidation, necessitating protective strategies .
- Byproduct formation : Over-oxidation to quinoline derivatives or decarboxylation under acidic conditions requires pH control (pH 4–6) and shorter reaction times .
- Catalyst selection : Transition metal catalysts (e.g., Cu²⁺ in copper salts) improve selectivity during crystallization .
Q. How can computational chemistry (e.g., quantum mechanical modeling) guide the design of novel synthetic routes for this compound?
Computational methods such as density functional theory (DFT) can predict reaction energetics and transition states. For example:
- Reaction path search : Identifying low-energy pathways for methyl group oxidation vs. ring hydroxylation.
- Solvent effects : Simulating polar protic solvents (e.g., water) to stabilize intermediates.
- Regioselectivity analysis : Mapping charge distribution to predict preferential substitution sites. Integrated computational-experimental frameworks, like those developed by ICReDD, enable rapid optimization by narrowing experimental conditions using quantum chemical calculations .
Q. How do steric and electronic effects influence the stability of this compound under varying pH and temperature conditions?
- Steric effects : The 6-methyl group may hinder protonation/deprotonation at the carboxylic acid group, increasing stability in acidic media.
- Electronic effects : Electron-donating hydroxyl groups enhance resonance stabilization of the carboxylate anion at neutral-to-basic pH.
- Thermal degradation : Accelerated decarboxylation above 100°C necessitates low-temperature storage and inert atmospheres. Experimental validation via thermogravimetric analysis (TGA) and pH-dependent stability studies is recommended.
Q. Methodological Notes
- Contradictions in evidence : While reports yields for methoxy analogs, direct data for the hydroxy-methyl variant is absent. Researchers should extrapolate from analogous systems and validate via controlled experiments.
- Advanced purification : Chromatographic techniques (e.g., HPLC with C18 columns) or recrystallization in ethanol/water mixtures can address purity challenges.
Comparación Con Compuestos Similares
Comparison with Structurally Similar Compounds
Substituted Pyridinecarboxylic Acids
Pyridinecarboxylic acids with varying substituents exhibit distinct physicochemical properties and reactivities. Below is a detailed comparison:
Table 1: Key Properties of 5-Hydroxy-6-methylpyridine-2-carboxylic Acid and Analogues
Compound Name | Substituents (Position) | Molecular Formula | Molecular Weight | PSA (Ų) | LogP | Melting Point (°C) | Synthetic Yield (%) |
---|---|---|---|---|---|---|---|
This compound | 5-OH, 6-CH₃, 2-COOH | C₇H₇NO₃ | 153.14 | 70.42 | 0.79 | N/A | N/A |
5-Methoxypyridine-2-carboxylic acid | 5-OCH₃, 2-COOH | C₇H₇NO₃ | 153.14 | N/A | N/A | 167 | 47 |
6-Methoxypyridine-2-carboxylic acid | 6-OCH₃, 2-COOH | C₇H₇NO₃ | 153.14 | N/A | N/A | 129–130 | 84 |
5-Cyano-6-methylpyridine-2-carboxylic acid | 5-CN, 6-CH₃, 2-COOH | C₈H₆N₂O₂ | 166.15 | N/A | N/A | N/A | N/A |
2-Hydroxy-6-methylpyridine-4-carboxylic acid | 2-OH, 6-CH₃, 4-COOH | C₇H₇NO₃ | 153.14 | N/A | N/A | N/A | N/A |
6-Methyl-2-pyridinecarboxylic acid | 6-CH₃, 2-COOH | C₇H₇NO₂ | 137.14 | N/A | N/A | N/A | N/A |
Key Observations :
Positional Isomerism :
- The target compound and 2-hydroxy-6-methylpyridine-4-carboxylic acid () share the same molecular formula but differ in substituent positions. The hydroxyl group at position 5 in the target compound enhances hydrogen-bonding capacity compared to the 2-hydroxy isomer, likely increasing solubility in polar solvents .
5-Cyano-6-methylpyridine-2-carboxylic acid () features a strongly electron-withdrawing cyano (-CN) group, which further enhances acidity compared to the hydroxyl substituent .
Synthetic Accessibility: Methoxy-substituted analogues (e.g., 5-methoxy and 6-methoxypyridine-2-carboxylic acids) are synthesized via oxidation of methylpyridines using KMnO₄, with yields ranging from 47% to 84% . The lower yield for the 5-methoxy derivative may reflect steric or electronic challenges during oxidation.
Physical Properties :
- The 5-methoxy derivative has a higher melting point (167°C) than the 6-methoxy isomer (129–130°C), suggesting differences in crystal packing due to substituent orientation .
Heterocyclic Analogues
Pyrrolo-Pyridine Derivatives ():
Compounds like 1H-pyrrolo[2,3-c]pyridine-2-carboxylic acid feature fused pyrrolo-pyridine rings, which introduce additional nitrogen atoms and alter electronic properties. These derivatives are synthesized in high yields (71–95%) but lack direct functional group comparability to the target compound .Pyrimidinecarboxylic Acids ():
4-Methyl-2-sulfanyl-5-pyrimidinecarboxylic acid (C₆H₆N₂O₂S) contains a pyrimidine core with a sulfur atom, leading to higher molecular weight (170.19 g/mol) and distinct reactivity compared to pyridine derivatives .
Propiedades
IUPAC Name |
5-hydroxy-6-methylpyridine-2-carboxylic acid | |
---|---|---|
Source | PubChem | |
URL | https://pubchem.ncbi.nlm.nih.gov | |
Description | Data deposited in or computed by PubChem | |
InChI |
InChI=1S/C7H7NO3/c1-4-6(9)3-2-5(8-4)7(10)11/h2-3,9H,1H3,(H,10,11) | |
Source | PubChem | |
URL | https://pubchem.ncbi.nlm.nih.gov | |
Description | Data deposited in or computed by PubChem | |
InChI Key |
KJGJZYTUFVQTNN-UHFFFAOYSA-N | |
Source | PubChem | |
URL | https://pubchem.ncbi.nlm.nih.gov | |
Description | Data deposited in or computed by PubChem | |
Canonical SMILES |
CC1=C(C=CC(=N1)C(=O)O)O | |
Source | PubChem | |
URL | https://pubchem.ncbi.nlm.nih.gov | |
Description | Data deposited in or computed by PubChem | |
Molecular Formula |
C7H7NO3 | |
Source | PubChem | |
URL | https://pubchem.ncbi.nlm.nih.gov | |
Description | Data deposited in or computed by PubChem | |
DSSTOX Substance ID |
DTXSID00300077 | |
Record name | 5-Hydroxy-6-methylpyridine-2-carboxylic acid | |
Source | EPA DSSTox | |
URL | https://comptox.epa.gov/dashboard/DTXSID00300077 | |
Description | DSSTox provides a high quality public chemistry resource for supporting improved predictive toxicology. | |
Molecular Weight |
153.14 g/mol | |
Source | PubChem | |
URL | https://pubchem.ncbi.nlm.nih.gov | |
Description | Data deposited in or computed by PubChem | |
CAS No. |
121447-41-4 | |
Record name | 5-Hydroxy-6-methylpyridine-2-carboxylic acid | |
Source | EPA DSSTox | |
URL | https://comptox.epa.gov/dashboard/DTXSID00300077 | |
Description | DSSTox provides a high quality public chemistry resource for supporting improved predictive toxicology. | |
Synthesis routes and methods
Procedure details
Descargo de responsabilidad e información sobre productos de investigación in vitro
Tenga en cuenta que todos los artículos e información de productos presentados en BenchChem están destinados únicamente con fines informativos. Los productos disponibles para la compra en BenchChem están diseñados específicamente para estudios in vitro, que se realizan fuera de organismos vivos. Los estudios in vitro, derivados del término latino "in vidrio", involucran experimentos realizados en entornos de laboratorio controlados utilizando células o tejidos. Es importante tener en cuenta que estos productos no se clasifican como medicamentos y no han recibido la aprobación de la FDA para la prevención, tratamiento o cura de ninguna condición médica, dolencia o enfermedad. Debemos enfatizar que cualquier forma de introducción corporal de estos productos en humanos o animales está estrictamente prohibida por ley. Es esencial adherirse a estas pautas para garantizar el cumplimiento de los estándares legales y éticos en la investigación y experimentación.