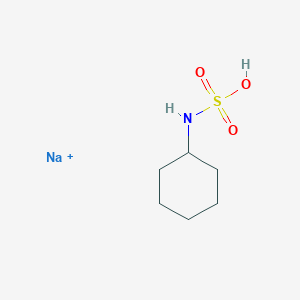
Sodium cyclamate
Descripción general
Descripción
Sodium cyclamate is an artificial sweetener that is 30 to 50 times sweeter than sugar . It is often used because of its taste, which is close to naturally occurring sugar . Sodium cyclamate is mainly used as a table-top sweetener, and as a non-medicinal ingredient in drugs .
Molecular Structure Analysis
Sodium cyclamate has the molecular formula C6H12NNaO3S . Its average mass is 201.219 Da and its monoisotopic mass is 201.043564 Da .Physical And Chemical Properties Analysis
Sodium cyclamate is a white, crystalline powder . It is stable to heat and readily soluble in water . It has a molecular weight of 201.22 and is soluble in water at a concentration of ≥ 100 mg/mL .Aplicaciones Científicas De Investigación
Watermelon Analysis Using LC-MS/MS
A study focused on estimating sodium cyclamate in watermelon using liquid chromatography-tandem mass spectrometry (LC-MS/MS) revealed the following:
- Results : The correlation coefficient for linearity was 0.999, with a detection limit of 1 ng/mL and a quantification limit of 3 ng/mL. Recovery was 88.5% at 200 ng/mL .
Photography Sensitizing Agents
Cyclamate has been employed as a sensitizing agent in color photography. While this application is less common, it highlights the compound’s versatility .
Soft Drinks and Beverages
Sodium cyclamate is still widely used as a sweetener in sugar-free soft drinks. Its non-caloric nature makes it suitable for this application .
Mecanismo De Acción
Target of Action
Sodium cyclamate, also known as cyclamic acid, is primarily used as an artificial sweetener . It targets the taste receptors on the tongue, specifically the sweet receptors, providing a sweet taste that is 30-50 times stronger than sucrose (table sugar) .
Mode of Action
Sodium cyclamate interacts with the sweet taste receptors on the tongue, triggering a response that the brain interprets as a sweet taste .
Biochemical Pathways
This metabolite has been a subject of concern due to its potential toxicity .
Pharmacokinetics
The compound that is absorbed is excreted unchanged by the kidneys . The plasma half-lives of cyclohexylamine, a possible metabolite of sodium cyclamate, range from 3.5 to 4.8 hours, showing a clear dose dependency .
Result of Action
The primary result of sodium cyclamate’s action is the perception of sweetness without the caloric content of sugar. This makes it a popular choice for use in diet foods and beverages, as well as in medications where it is used to mask bitterness . There have been concerns about its safety due to the potential toxicity of its metabolite, cyclohexylamine .
Action Environment
Sodium cyclamate is stable under heat, making it suitable for use in cooking and baking . . For example, it is often used in combination with other artificial sweeteners, such as saccharin, to mask the off-tastes of both sweeteners .
Direcciones Futuras
An analysis of information related to current and potential future uses of sodium cyclamate suggests that it is unlikely that exposure will increase to levels of concern to human health . For this reason, follow-up activities to track changes in exposure and/or commercial use patterns for sodium cyclamate are not being considered at this time .
Propiedades
IUPAC Name |
sodium;N-cyclohexylsulfamate | |
---|---|---|
Source | PubChem | |
URL | https://pubchem.ncbi.nlm.nih.gov | |
Description | Data deposited in or computed by PubChem | |
InChI |
InChI=1S/C6H13NO3S.Na/c8-11(9,10)7-6-4-2-1-3-5-6;/h6-7H,1-5H2,(H,8,9,10);/q;+1/p-1 | |
Source | PubChem | |
URL | https://pubchem.ncbi.nlm.nih.gov | |
Description | Data deposited in or computed by PubChem | |
InChI Key |
UDIPTWFVPPPURJ-UHFFFAOYSA-M | |
Source | PubChem | |
URL | https://pubchem.ncbi.nlm.nih.gov | |
Description | Data deposited in or computed by PubChem | |
Canonical SMILES |
C1CCC(CC1)NS(=O)(=O)[O-].[Na+] | |
Source | PubChem | |
URL | https://pubchem.ncbi.nlm.nih.gov | |
Description | Data deposited in or computed by PubChem | |
Molecular Formula |
C6H11NHSO3.Na, C6H12NNaO3S | |
Record name | SODIUM CYCLAMATE | |
Source | CAMEO Chemicals | |
URL | https://cameochemicals.noaa.gov/chemical/21017 | |
Description | CAMEO Chemicals is a chemical database designed for people who are involved in hazardous material incident response and planning. CAMEO Chemicals contains a library with thousands of datasheets containing response-related information and recommendations for hazardous materials that are commonly transported, used, or stored in the United States. CAMEO Chemicals was developed by the National Oceanic and Atmospheric Administration's Office of Response and Restoration in partnership with the Environmental Protection Agency's Office of Emergency Management. | |
Explanation | CAMEO Chemicals and all other CAMEO products are available at no charge to those organizations and individuals (recipients) responsible for the safe handling of chemicals. However, some of the chemical data itself is subject to the copyright restrictions of the companies or organizations that provided the data. | |
Source | PubChem | |
URL | https://pubchem.ncbi.nlm.nih.gov | |
Description | Data deposited in or computed by PubChem | |
DSSTOX Substance ID |
DTXSID6020355 | |
Record name | Sodium cyclamate | |
Source | EPA DSSTox | |
URL | https://comptox.epa.gov/dashboard/DTXSID6020355 | |
Description | DSSTox provides a high quality public chemistry resource for supporting improved predictive toxicology. | |
Molecular Weight |
201.22 g/mol | |
Source | PubChem | |
URL | https://pubchem.ncbi.nlm.nih.gov | |
Description | Data deposited in or computed by PubChem | |
Physical Description |
Sodium cyclamate appears as odorless or almost odorless white crystals or crystalline powder. Intensely sweet taste, even in dilute solution. pH (10% solution in water): 5.5-7.5. Used as a non-nutritive sweetener., White solid; [Hawley] White powder; [MSDSonline] | |
Record name | SODIUM CYCLAMATE | |
Source | CAMEO Chemicals | |
URL | https://cameochemicals.noaa.gov/chemical/21017 | |
Description | CAMEO Chemicals is a chemical database designed for people who are involved in hazardous material incident response and planning. CAMEO Chemicals contains a library with thousands of datasheets containing response-related information and recommendations for hazardous materials that are commonly transported, used, or stored in the United States. CAMEO Chemicals was developed by the National Oceanic and Atmospheric Administration's Office of Response and Restoration in partnership with the Environmental Protection Agency's Office of Emergency Management. | |
Explanation | CAMEO Chemicals and all other CAMEO products are available at no charge to those organizations and individuals (recipients) responsible for the safe handling of chemicals. However, some of the chemical data itself is subject to the copyright restrictions of the companies or organizations that provided the data. | |
Record name | Sodium cyclamate | |
Source | Haz-Map, Information on Hazardous Chemicals and Occupational Diseases | |
URL | https://haz-map.com/Agents/7864 | |
Description | Haz-Map® is an occupational health database designed for health and safety professionals and for consumers seeking information about the adverse effects of workplace exposures to chemical and biological agents. | |
Explanation | Copyright (c) 2022 Haz-Map(R). All rights reserved. Unless otherwise indicated, all materials from Haz-Map are copyrighted by Haz-Map(R). No part of these materials, either text or image may be used for any purpose other than for personal use. Therefore, reproduction, modification, storage in a retrieval system or retransmission, in any form or by any means, electronic, mechanical or otherwise, for reasons other than personal use, is strictly prohibited without prior written permission. | |
Solubility |
greater than or equal to 100 mg/mL at 68 °F (NTP, 1992) | |
Record name | SODIUM CYCLAMATE | |
Source | CAMEO Chemicals | |
URL | https://cameochemicals.noaa.gov/chemical/21017 | |
Description | CAMEO Chemicals is a chemical database designed for people who are involved in hazardous material incident response and planning. CAMEO Chemicals contains a library with thousands of datasheets containing response-related information and recommendations for hazardous materials that are commonly transported, used, or stored in the United States. CAMEO Chemicals was developed by the National Oceanic and Atmospheric Administration's Office of Response and Restoration in partnership with the Environmental Protection Agency's Office of Emergency Management. | |
Explanation | CAMEO Chemicals and all other CAMEO products are available at no charge to those organizations and individuals (recipients) responsible for the safe handling of chemicals. However, some of the chemical data itself is subject to the copyright restrictions of the companies or organizations that provided the data. | |
Product Name |
Sodium cyclamate | |
CAS RN |
139-05-9 | |
Record name | SODIUM CYCLAMATE | |
Source | CAMEO Chemicals | |
URL | https://cameochemicals.noaa.gov/chemical/21017 | |
Description | CAMEO Chemicals is a chemical database designed for people who are involved in hazardous material incident response and planning. CAMEO Chemicals contains a library with thousands of datasheets containing response-related information and recommendations for hazardous materials that are commonly transported, used, or stored in the United States. CAMEO Chemicals was developed by the National Oceanic and Atmospheric Administration's Office of Response and Restoration in partnership with the Environmental Protection Agency's Office of Emergency Management. | |
Explanation | CAMEO Chemicals and all other CAMEO products are available at no charge to those organizations and individuals (recipients) responsible for the safe handling of chemicals. However, some of the chemical data itself is subject to the copyright restrictions of the companies or organizations that provided the data. | |
Record name | Sodium cyclamate [INN:BAN:NF] | |
Source | ChemIDplus | |
URL | https://pubchem.ncbi.nlm.nih.gov/substance/?source=chemidplus&sourceid=0000139059 | |
Description | ChemIDplus is a free, web search system that provides access to the structure and nomenclature authority files used for the identification of chemical substances cited in National Library of Medicine (NLM) databases, including the TOXNET system. | |
Record name | Sulfamic acid, N-cyclohexyl-, sodium salt (1:1) | |
Source | EPA Chemicals under the TSCA | |
URL | https://www.epa.gov/chemicals-under-tsca | |
Description | EPA Chemicals under the Toxic Substances Control Act (TSCA) collection contains information on chemicals and their regulations under TSCA, including non-confidential content from the TSCA Chemical Substance Inventory and Chemical Data Reporting. | |
Record name | Sodium cyclamate | |
Source | EPA DSSTox | |
URL | https://comptox.epa.gov/dashboard/DTXSID6020355 | |
Description | DSSTox provides a high quality public chemistry resource for supporting improved predictive toxicology. | |
Record name | Sodium cyclamate | |
Source | European Chemicals Agency (ECHA) | |
URL | https://echa.europa.eu/substance-information/-/substanceinfo/100.004.863 | |
Description | The European Chemicals Agency (ECHA) is an agency of the European Union which is the driving force among regulatory authorities in implementing the EU's groundbreaking chemicals legislation for the benefit of human health and the environment as well as for innovation and competitiveness. | |
Explanation | Use of the information, documents and data from the ECHA website is subject to the terms and conditions of this Legal Notice, and subject to other binding limitations provided for under applicable law, the information, documents and data made available on the ECHA website may be reproduced, distributed and/or used, totally or in part, for non-commercial purposes provided that ECHA is acknowledged as the source: "Source: European Chemicals Agency, http://echa.europa.eu/". Such acknowledgement must be included in each copy of the material. ECHA permits and encourages organisations and individuals to create links to the ECHA website under the following cumulative conditions: Links can only be made to webpages that provide a link to the Legal Notice page. | |
Record name | SODIUM CYCLAMATE | |
Source | FDA Global Substance Registration System (GSRS) | |
URL | https://gsrs.ncats.nih.gov/ginas/app/beta/substances/1I6F42RME1 | |
Description | The FDA Global Substance Registration System (GSRS) enables the efficient and accurate exchange of information on what substances are in regulated products. Instead of relying on names, which vary across regulatory domains, countries, and regions, the GSRS knowledge base makes it possible for substances to be defined by standardized, scientific descriptions. | |
Explanation | Unless otherwise noted, the contents of the FDA website (www.fda.gov), both text and graphics, are not copyrighted. They are in the public domain and may be republished, reprinted and otherwise used freely by anyone without the need to obtain permission from FDA. Credit to the U.S. Food and Drug Administration as the source is appreciated but not required. | |
Melting Point |
509 °F (decomposes) (NTP, 1992) | |
Record name | SODIUM CYCLAMATE | |
Source | CAMEO Chemicals | |
URL | https://cameochemicals.noaa.gov/chemical/21017 | |
Description | CAMEO Chemicals is a chemical database designed for people who are involved in hazardous material incident response and planning. CAMEO Chemicals contains a library with thousands of datasheets containing response-related information and recommendations for hazardous materials that are commonly transported, used, or stored in the United States. CAMEO Chemicals was developed by the National Oceanic and Atmospheric Administration's Office of Response and Restoration in partnership with the Environmental Protection Agency's Office of Emergency Management. | |
Explanation | CAMEO Chemicals and all other CAMEO products are available at no charge to those organizations and individuals (recipients) responsible for the safe handling of chemicals. However, some of the chemical data itself is subject to the copyright restrictions of the companies or organizations that provided the data. | |
Retrosynthesis Analysis
AI-Powered Synthesis Planning: Our tool employs the Template_relevance Pistachio, Template_relevance Bkms_metabolic, Template_relevance Pistachio_ringbreaker, Template_relevance Reaxys, Template_relevance Reaxys_biocatalysis model, leveraging a vast database of chemical reactions to predict feasible synthetic routes.
One-Step Synthesis Focus: Specifically designed for one-step synthesis, it provides concise and direct routes for your target compounds, streamlining the synthesis process.
Accurate Predictions: Utilizing the extensive PISTACHIO, BKMS_METABOLIC, PISTACHIO_RINGBREAKER, REAXYS, REAXYS_BIOCATALYSIS database, our tool offers high-accuracy predictions, reflecting the latest in chemical research and data.
Strategy Settings
Precursor scoring | Relevance Heuristic |
---|---|
Min. plausibility | 0.01 |
Model | Template_relevance |
Template Set | Pistachio/Bkms_metabolic/Pistachio_ringbreaker/Reaxys/Reaxys_biocatalysis |
Top-N result to add to graph | 6 |
Feasible Synthetic Routes
Descargo de responsabilidad e información sobre productos de investigación in vitro
Tenga en cuenta que todos los artículos e información de productos presentados en BenchChem están destinados únicamente con fines informativos. Los productos disponibles para la compra en BenchChem están diseñados específicamente para estudios in vitro, que se realizan fuera de organismos vivos. Los estudios in vitro, derivados del término latino "in vidrio", involucran experimentos realizados en entornos de laboratorio controlados utilizando células o tejidos. Es importante tener en cuenta que estos productos no se clasifican como medicamentos y no han recibido la aprobación de la FDA para la prevención, tratamiento o cura de ninguna condición médica, dolencia o enfermedad. Debemos enfatizar que cualquier forma de introducción corporal de estos productos en humanos o animales está estrictamente prohibida por ley. Es esencial adherirse a estas pautas para garantizar el cumplimiento de los estándares legales y éticos en la investigación y experimentación.