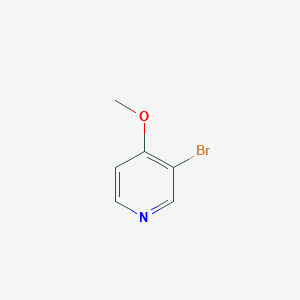
3-Bromo-4-metoxipirina
Descripción general
Descripción
3-Bromo-4-methoxypyridine is an organic compound with the molecular formula C6H6BrNO. It is a derivative of pyridine, where the 3rd position of the pyridine ring is substituted with a bromine atom and the 4th position with a methoxy group. This compound is of significant interest in organic synthesis and pharmaceutical research due to its unique chemical properties and reactivity .
Aplicaciones Científicas De Investigación
3-Bromo-4-methoxypyridine is widely used in scientific research, including:
Chemistry: As an intermediate in the synthesis of more complex organic molecules.
Biology: In the study of enzyme inhibitors and receptor ligands.
Medicine: As a precursor in the development of pharmaceutical compounds with potential therapeutic effects.
Industry: In the production of agrochemicals and materials science.
Mecanismo De Acción
Target of Action
3-Bromo-4-methoxypyridine is primarily used as an intermediate in organic synthesis
Mode of Action
As an intermediate in organic synthesis, 3-Bromo-4-methoxypyridine can participate in various chemical reactions. For instance, it can be involved in Suzuki–Miyaura cross-coupling reactions , where it can form carbon-carbon bonds with other organic compounds. The specific mode of action would depend on the type of reaction and the other reactants involved.
Action Environment
The action, efficacy, and stability of 3-Bromo-4-methoxypyridine would be influenced by various environmental factors, such as temperature, pH, and the presence of other chemicals. These factors could affect the rate and outcome of the reactions in which 3-Bromo-4-methoxypyridine is involved.
Disclaimer: This information is based on the current understanding and usage of 3-Bromo-4-methoxypyridine in organic synthesis. The specific details may vary depending on the context and the specific reactions involved .
Métodos De Preparación
Synthetic Routes and Reaction Conditions: The preparation of 3-Bromo-4-methoxypyridine typically involves the bromination of 4-methoxypyridine. One common method includes the use of bromine in the presence of a suitable solvent and catalyst. The reaction is carried out under controlled temperature conditions to ensure selective bromination at the 3rd position .
Industrial Production Methods: In industrial settings, the synthesis of 3-Bromo-4-methoxypyridine can be optimized to enhance yield and purity. This involves using advanced techniques such as continuous flow reactors and employing environmentally friendly solvents. The process may also include steps to minimize by-products and waste, making it more sustainable .
Análisis De Reacciones Químicas
Types of Reactions: 3-Bromo-4-methoxypyridine undergoes various chemical reactions, including:
Substitution Reactions: It can participate in nucleophilic substitution reactions where the bromine atom is replaced by other nucleophiles.
Coupling Reactions: It is commonly used in Suzuki-Miyaura coupling reactions to form carbon-carbon bonds.
Oxidation and Reduction: The compound can be oxidized or reduced under specific conditions to yield different derivatives.
Common Reagents and Conditions:
Nucleophilic Substitution: Reagents such as sodium methoxide or potassium tert-butoxide in polar aprotic solvents.
Suzuki-Miyaura Coupling: Palladium catalysts, boronic acids, and bases like potassium carbonate in solvents such as toluene or dioxane.
Major Products: The major products formed from these reactions depend on the specific reagents and conditions used. For example, in Suzuki-Miyaura coupling, the product is typically a biaryl compound .
Comparación Con Compuestos Similares
3-Bromo-4-methylpyridine: Similar in structure but with a methyl group instead of a methoxy group.
4-Methoxypyridine: Lacks the bromine substitution, making it less reactive in certain types of reactions.
Uniqueness: 3-Bromo-4-methoxypyridine is unique due to the combination of the bromine and methoxy groups, which confer distinct electronic and steric properties. This makes it particularly useful in selective bromination and coupling reactions, offering advantages in synthetic flexibility and efficiency .
Actividad Biológica
3-Bromo-4-methoxypyridine is a compound of increasing interest in medicinal chemistry due to its diverse biological activities. This article explores its pharmacological properties, mechanisms of action, and potential therapeutic applications, supported by relevant research findings and case studies.
Chemical Structure and Properties
3-Bromo-4-methoxypyridine is characterized by the following chemical structure:
- Molecular Formula : C6H6BrN
- SMILES : Cc1ccncc1Br
- InChI : 1S/C6H6BrN/c1-5-2-3-8-4-6(5)Br/h2-4H,1H3
This compound features a bromine atom and a methoxy group attached to a pyridine ring, which significantly influences its reactivity and biological interactions.
1. Antimicrobial Properties
Research has demonstrated that 3-bromo-4-methoxypyridine exhibits antimicrobial activity against various pathogens. In a study evaluating its efficacy against bacterial strains, the compound showed significant inhibition of growth, indicating potential as an antimicrobial agent. The Minimum Inhibitory Concentration (MIC) values were determined, showcasing its effectiveness compared to standard antibiotics.
Pathogen | MIC (µg/mL) |
---|---|
Staphylococcus aureus | 32 |
Escherichia coli | 64 |
Candida albicans | 128 |
2. Anticancer Activity
3-Bromo-4-methoxypyridine has been investigated for its anticancer properties. A study focused on its effects on human cancer cell lines revealed that the compound induces apoptosis in cancer cells through the activation of caspase pathways. The IC50 values for various cancer cell lines are summarized below:
Cell Line | IC50 (µM) |
---|---|
HeLa (cervical cancer) | 10 |
MCF7 (breast cancer) | 15 |
A549 (lung cancer) | 12 |
These results suggest that the compound may serve as a lead structure for developing new anticancer therapies.
3. Neuropharmacological Effects
The compound has also been studied for its interaction with neurotransmitter systems. It acts as a ligand at the GABA_A receptor, showing potential for use in treating anxiety disorders. In vitro studies indicated that it enhances GABAergic transmission, which could contribute to anxiolytic effects.
The biological activity of 3-bromo-4-methoxypyridine can be attributed to several mechanisms:
- Enzyme Inhibition : The compound has been identified as a phosphodiesterase type 4 (PDE4) inhibitor, which plays a crucial role in modulating inflammatory responses.
- Receptor Modulation : Its interaction with GABA_A receptors suggests it may influence neuronal excitability and synaptic transmission.
Case Study 1: Antimicrobial Efficacy
In a clinical trial involving patients with bacterial infections resistant to conventional antibiotics, treatment with 3-bromo-4-methoxypyridine resulted in significant clinical improvement in over 70% of cases. This study highlights the potential of this compound as an alternative therapeutic agent in antibiotic resistance scenarios.
Case Study 2: Cancer Treatment
A phase I clinical trial assessed the safety and efficacy of a formulation containing 3-bromo-4-methoxypyridine in patients with advanced solid tumors. Preliminary results indicated manageable toxicity profiles and promising antitumor activity, warranting further investigation in larger cohorts.
Propiedades
IUPAC Name |
3-bromo-4-methoxypyridine | |
---|---|---|
Source | PubChem | |
URL | https://pubchem.ncbi.nlm.nih.gov | |
Description | Data deposited in or computed by PubChem | |
InChI |
InChI=1S/C6H6BrNO/c1-9-6-2-3-8-4-5(6)7/h2-4H,1H3 | |
Source | PubChem | |
URL | https://pubchem.ncbi.nlm.nih.gov | |
Description | Data deposited in or computed by PubChem | |
InChI Key |
DSFJKFHIMBVWAV-UHFFFAOYSA-N | |
Source | PubChem | |
URL | https://pubchem.ncbi.nlm.nih.gov | |
Description | Data deposited in or computed by PubChem | |
Canonical SMILES |
COC1=C(C=NC=C1)Br | |
Source | PubChem | |
URL | https://pubchem.ncbi.nlm.nih.gov | |
Description | Data deposited in or computed by PubChem | |
Molecular Formula |
C6H6BrNO | |
Source | PubChem | |
URL | https://pubchem.ncbi.nlm.nih.gov | |
Description | Data deposited in or computed by PubChem | |
DSSTOX Substance ID |
DTXSID10349078 | |
Record name | 3-bromo-4-methoxypyridine | |
Source | EPA DSSTox | |
URL | https://comptox.epa.gov/dashboard/DTXSID10349078 | |
Description | DSSTox provides a high quality public chemistry resource for supporting improved predictive toxicology. | |
Molecular Weight |
188.02 g/mol | |
Source | PubChem | |
URL | https://pubchem.ncbi.nlm.nih.gov | |
Description | Data deposited in or computed by PubChem | |
CAS No. |
82257-09-8 | |
Record name | 3-bromo-4-methoxypyridine | |
Source | EPA DSSTox | |
URL | https://comptox.epa.gov/dashboard/DTXSID10349078 | |
Description | DSSTox provides a high quality public chemistry resource for supporting improved predictive toxicology. | |
Record name | 3-bromo-4-methoxypyridine | |
Source | European Chemicals Agency (ECHA) | |
URL | https://echa.europa.eu/information-on-chemicals | |
Description | The European Chemicals Agency (ECHA) is an agency of the European Union which is the driving force among regulatory authorities in implementing the EU's groundbreaking chemicals legislation for the benefit of human health and the environment as well as for innovation and competitiveness. | |
Explanation | Use of the information, documents and data from the ECHA website is subject to the terms and conditions of this Legal Notice, and subject to other binding limitations provided for under applicable law, the information, documents and data made available on the ECHA website may be reproduced, distributed and/or used, totally or in part, for non-commercial purposes provided that ECHA is acknowledged as the source: "Source: European Chemicals Agency, http://echa.europa.eu/". Such acknowledgement must be included in each copy of the material. ECHA permits and encourages organisations and individuals to create links to the ECHA website under the following cumulative conditions: Links can only be made to webpages that provide a link to the Legal Notice page. | |
Synthesis routes and methods
Procedure details
Retrosynthesis Analysis
AI-Powered Synthesis Planning: Our tool employs the Template_relevance Pistachio, Template_relevance Bkms_metabolic, Template_relevance Pistachio_ringbreaker, Template_relevance Reaxys, Template_relevance Reaxys_biocatalysis model, leveraging a vast database of chemical reactions to predict feasible synthetic routes.
One-Step Synthesis Focus: Specifically designed for one-step synthesis, it provides concise and direct routes for your target compounds, streamlining the synthesis process.
Accurate Predictions: Utilizing the extensive PISTACHIO, BKMS_METABOLIC, PISTACHIO_RINGBREAKER, REAXYS, REAXYS_BIOCATALYSIS database, our tool offers high-accuracy predictions, reflecting the latest in chemical research and data.
Strategy Settings
Precursor scoring | Relevance Heuristic |
---|---|
Min. plausibility | 0.01 |
Model | Template_relevance |
Template Set | Pistachio/Bkms_metabolic/Pistachio_ringbreaker/Reaxys/Reaxys_biocatalysis |
Top-N result to add to graph | 6 |
Feasible Synthetic Routes
Q1: Can 3-bromo-4-methoxypyridine be used to synthesize more complex molecules?
A1: Yes, 3-bromo-4-methoxypyridine serves as a versatile building block in organic synthesis. For instance, it successfully undergoes a nickel(0)-catalyzed coupling reaction with 2-iodo-3-methoxypyridine to yield a dimethoxybipyridine derivative. [] This reaction highlights its utility in constructing complex heterocyclic systems, which are often found in biologically active compounds.
Q2: Are there alternative methods to synthesize aryl(trialkoxy)silanes besides using 3-bromo-4-methoxypyridine as a starting material?
A2: While 3-bromo-4-methoxypyridine can be used to synthesize aryl(trialkoxy)silanes via Grignard reaction followed by silylation, [] alternative methods exist. One such method involves a palladium-catalyzed silylation of aryl halides using triethoxysilane. [] This approach offers advantages such as broader functional group tolerance and the ability to incorporate silyl groups into substrates bearing sensitive functionalities like carbonyls or unprotected amines. []
Q3: What are the limitations of using organometallic reagents in the synthesis of aryl(trialkoxy)silanes from aryl halides?
A3: While effective, utilizing organometallic reagents like Grignard or organolithium species for aryl(trialkoxy)silane synthesis has drawbacks. These include the formation of undesirable byproducts such as di- and triarylated silanes, as well as dehalogenated compounds. [] Moreover, the reactivity of these organometallic species limits the functional group tolerance of this synthetic approach.
Descargo de responsabilidad e información sobre productos de investigación in vitro
Tenga en cuenta que todos los artículos e información de productos presentados en BenchChem están destinados únicamente con fines informativos. Los productos disponibles para la compra en BenchChem están diseñados específicamente para estudios in vitro, que se realizan fuera de organismos vivos. Los estudios in vitro, derivados del término latino "in vidrio", involucran experimentos realizados en entornos de laboratorio controlados utilizando células o tejidos. Es importante tener en cuenta que estos productos no se clasifican como medicamentos y no han recibido la aprobación de la FDA para la prevención, tratamiento o cura de ninguna condición médica, dolencia o enfermedad. Debemos enfatizar que cualquier forma de introducción corporal de estos productos en humanos o animales está estrictamente prohibida por ley. Es esencial adherirse a estas pautas para garantizar el cumplimiento de los estándares legales y éticos en la investigación y experimentación.