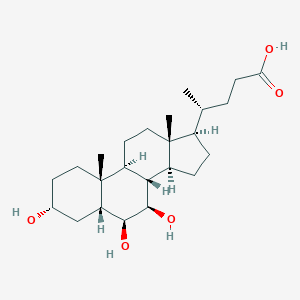
beta-Muricholic acid
Descripción general
Descripción
Beta-muricholic acid (beta-MCA) is a trihydroxy bile acid with the chemical structure 3α,6β,7β-trihydroxy-5β-cholanic acid . It is a hydrophilic bile acid naturally synthesized in the livers of rodents, including mice, rats, and hamsters . Beta-MCA plays critical roles in cholesterol homeostasis, gallstone prevention, and bile acid metabolism. Its hydrophilicity distinguishes it from more hydrophobic bile acids like chenodeoxycholic acid (CDCA) and contributes to its unique physiological effects, such as reducing biliary cholesterol saturation and preventing crystallization .
Métodos De Preparación
Synthetic Routes and Reaction Conditions: Beta-Muricholic acid can be synthesized from ursodeoxycholic acid through a hydroxylation reaction at the 6-position. The enzyme cytochrome P450 Cyp2c70 is responsible for this conversion in rodents . The reaction conditions typically involve the presence of this enzyme and suitable cofactors to facilitate the hydroxylation process.
Industrial Production Methods: Industrial production of this compound involves the extraction and purification from bile sources, particularly from rodents. The process includes several steps such as bile collection, extraction, purification, and crystallization to obtain the compound in its pure form .
Análisis De Reacciones Químicas
Types of Reactions: Beta-Muricholic acid undergoes various chemical reactions, including:
Oxidation: this compound can be oxidized to form different oxo derivatives.
Reduction: Reduction reactions can convert oxo derivatives back to this compound.
Substitution: Hydroxyl groups in this compound can undergo substitution reactions to form various derivatives.
Common Reagents and Conditions:
Oxidation: Common oxidizing agents include potassium permanganate and chromium trioxide.
Reduction: Reducing agents like sodium borohydride and lithium aluminum hydride are used.
Substitution: Reagents such as acyl chlorides and alkyl halides are employed for substitution reactions.
Major Products:
Oxidation: Formation of oxo derivatives.
Reduction: Regeneration of this compound from oxo derivatives.
Substitution: Formation of acylated or alkylated derivatives of this compound.
Aplicaciones Científicas De Investigación
Cholesterol Gallstone Prevention and Treatment
Overview
Research indicates that β-MCA plays a significant role in preventing and dissolving cholesterol gallstones. A study conducted on male C57L mice demonstrated that β-MCA was more effective than ursodeoxycholic acid (UDCA) in both preventing the formation of cholesterol gallstones and facilitating their dissolution. Mice fed a lithogenic diet supplemented with β-MCA showed a gallstone prevalence reduction to 20%, compared to 50% with UDCA, highlighting its superior efficacy in managing cholesterol levels and promoting bile acid homeostasis .
Mechanism of Action
The mechanism by which β-MCA exerts its effects involves reducing the biliary secretion rate and saturation index of cholesterol, as well as enhancing the phase boundary shift that prevents cholesterol crystallization. This action leads to a significant decrease in gallstone formation and promotes complete dissolution rates when administered over eight weeks .
Liver Fibrosis and Gut Barrier Function Improvement
Recent Findings
Recent studies have identified β-MCA's potential in improving liver fibrosis and gut barrier function. In experiments involving Cyp2c70 knockout mice, which simulate human-like hydrophobic bile acid-induced hepatobiliary injury, treatment with glycine-conjugated β-MCA (G-β-MCA) resulted in reduced liver fibrosis and enhanced gut barrier integrity. The treatment led to decreased bile acid hydrophobicity and an increase in fecal bile acid excretion, suggesting a protective role against cholestasis-related liver damage .
FXR Antagonism and Metabolic Regulation
Role as an FXR Antagonist
β-MCA functions as an antagonist of the farnesoid X receptor (FXR), a key regulator of bile acid synthesis and metabolism. By modulating FXR signaling pathways, β-MCA influences glucose homeostasis and lipid metabolism, offering potential therapeutic avenues for metabolic disorders. Its ability to regulate bile acid synthesis without endocrine effects has been highlighted as crucial for understanding bile acid metabolism in both mice and humans .
Impact on Gut Microbiota
Modulation of Gut Microbiota
The interaction between β-MCA and gut microbiota is another area of interest. Studies suggest that β-MCA may alter the composition of gut microbiota, which can have downstream effects on metabolic health and disease states. The modulation of gut bacteria through bile acids like β-MCA can influence systemic inflammation and metabolic syndromes .
Data Summary Table
Case Studies
- Cholesterol Gallstones : In a controlled study with C57L mice, β-MCA administration resulted in a marked decrease in cholesterol gallstone formation compared to controls, demonstrating its effectiveness as a preventive treatment .
- Liver Health : In Cyp2c70 knockout mice treated with G-β-MCA, significant improvements in liver fibrosis markers were observed alongside enhanced gut barrier function, indicating its potential for treating liver diseases associated with cholestasis .
- Metabolic Regulation : Studies exploring the role of β-MCA as an FXR antagonist have shown promising results in regulating lipid metabolism pathways, suggesting therapeutic implications for conditions like obesity and diabetes .
Mecanismo De Acción
Beta-Muricholic acid exerts its effects primarily through interaction with bile acid receptors such as the farnesoid X receptor (FXR). It acts as an antagonist to FXR, thereby regulating bile acid synthesis and metabolism. The compound also influences lipid metabolism by inhibiting lipid accumulation in hepatocytes . The molecular targets include enzymes involved in bile acid synthesis and transporters responsible for bile acid reabsorption .
Comparación Con Compuestos Similares
Beta-Muricholic Acid vs. Ursodeoxycholic Acid (UDCA)
Mechanistic Differences :
- Gallstone Prevention & Dissolution :
- In C57L/J mice fed a lithogenic diet, beta-MCA reduced gallstone prevalence to 20% (vs. 50% with UDCA) by lowering biliary cholesterol secretion, saturation index, and intestinal cholesterol absorption .
- Beta-MCA achieved a 100% gallstone dissolution rate after 8 weeks, compared to 60% with UDCA, likely due to its ability to shift cholesterol from solid crystals to liquid crystalline phases .
- Physicochemical Properties: Beta-MCA has a lower monomer solubility (13 µM) and higher equilibrium precipitation pH (7.92) than UDCA, limiting its micellar capacity but enhancing cholesterol stabilization in non-micellar aggregates .
Table 1: Key Differences Between Beta-MCA and UDCA
This compound vs. Chenodeoxycholic Acid (CDCA)
Functional Contrast :
- Cholesterol Solubilization :
- Metabolic Pathways :
Table 2: Beta-MCA vs. CDCA
This compound vs. Other Muricholic Acids
Alpha-Muricholic Acid (alpha-MCA) and Gamma-Muricholic Acid (gamma-MCA) :
- Structural Differences :
- Beta-MCA is synthesized at 3-fold higher rates than cholic acid in rat hepatocyte cultures, unlike alpha-MCA .
Table 3: Muricholic Acid Isoforms
Species-Specific Metabolism
- Rodents vs. Humans :
Research Implications and Limitations
- Mechanistic Insights : Beta-MCA’s efficacy in gallstone prevention stems from phase boundary modulation rather than cholesterol solubilization .
- Translational Challenges : Rodent-specific synthesis limits direct human applications, though insights into hydrophilicity and crystallization inhibition inform drug design .
Actividad Biológica
Beta-muricholic acid (β-MCA) is a bile acid predominantly found in rodents, recognized for its significant biological activities, particularly in the regulation of bile acid metabolism, cholesterol homeostasis, and liver health. This article explores the biological activity of β-MCA through various studies, highlighting its mechanisms, effects on gallstone formation, and interactions with gut microbiota.
Overview of this compound
This compound is a trihydroxy bile acid that serves as an important regulator of cholesterol metabolism and bile acid synthesis. It has been shown to exert various physiological effects, including modulation of liver function and gut microbiota interactions.
Regulation of Bile Acid Synthesis
Research indicates that β-MCA plays a crucial role in the feedback regulation of bile acid synthesis. In studies involving Cyp8b1 knockout mice, β-MCA was identified as an FXR (farnesoid X receptor) antagonist that influences bile acid metabolism by reducing the levels of FXR-agonistic bile acids such as cholic acid (CA) and chenodeoxycholic acid (CDCA) . This antagonistic action leads to increased synthesis of bile acids through a positive feedback mechanism.
Effects on Cholesterol Gallstones
This compound has been shown to be effective in preventing and dissolving cholesterol gallstones. In experimental models, β-MCA demonstrated superior efficacy compared to ursodeoxycholic acid (UDCA). Mice fed a lithogenic diet exhibited gallstone formation; however, the introduction of β-MCA reduced gallstone prevalence significantly by affecting biliary cholesterol saturation and absorption .
Study on Gallstone Prevention
A pivotal study investigated the effects of β-MCA on cholesterol gallstone formation in male C57L mice. The results indicated that:
- Gallstone Formation : 100% of control mice formed gallstones on a lithogenic diet.
- Prevention Rates : Mice treated with β-MCA showed a reduction in gallstone prevalence to 20%, compared to 50% for UDCA.
- Mechanism : The treatment resulted in decreased biliary secretion rates and intestinal cholesterol absorption, facilitating phase boundary shifts that prevent solid crystal formation .
Metabolism and Excretion Patterns
In human studies involving patients post-cholecystectomy, metabolites of β-MCA were analyzed in bile, urine, and feces. The findings revealed that glyco- and tauro-β-MCA were the primary metabolites detected in bile, while the urinary pattern included free and conjugated forms of β-MCA . This suggests limited transformation by human intestinal microbiota, highlighting species-specific metabolic pathways.
Interaction with Gut Microbiota
The gut microbiota significantly influences the metabolism of bile acids, including β-MCA. Studies have shown that:
- Microbial Regulation : The gut microbiome can modulate the levels of secondary bile acids derived from β-MCA, impacting overall metabolic health .
- Health Correlations : In human subjects, specific bile acids correlated with metabolic markers such as body mass index (BMI) and insulin resistance, indicating potential roles for β-MCA in metabolic regulation .
Summary Table: Biological Activity of this compound
Q & A
Q. What are the standard analytical methods for quantifying β-Muricholic Acid in biological samples, and how do they address sensitivity and specificity?
Basic Research Focus
β-MCA quantification typically employs liquid chromatography-mass spectrometry (LC-MS) with multiple reaction monitoring (MRM) due to its high specificity for bile acids. For example, LC-MS protocols often use C18 reversed-phase columns and mobile phases optimized with formic acid (0.1%) to enhance ionization . To mitigate matrix effects, isotopically labeled internal standards (e.g., deuterated β-MCA) are recommended for normalization . HPLC with UV/Vis detection is less common due to lower sensitivity but may be used for preliminary screening .
Q. How does β-MCA’s hydroxylation pattern influence its interaction with bile acid receptors (e.g., FXR, TGR5), and what experimental models are optimal for studying these interactions?
Advanced Research Focus
β-MCA’s 3α,6β,7β-trihydroxy structure confers unique receptor-binding properties. Unlike cholic acid, β-MCA acts as a weak FXR antagonist but may modulate TGR5 signaling in intestinal L-cells. In vitro models include:
- HEK293 cells transfected with FXR or TGR5 reporters to measure luciferase activity.
- Primary hepatocyte cultures to assess gene expression (e.g., CYP7A1) under β-MCA treatment .
In vivo, bile acid receptor knockout mice (e.g., Fxr⁻/⁻) fed β-MCA-supplemented diets (0.5% w/w) are used to study metabolic outcomes over 8–12 weeks .
Q. What experimental designs are recommended to resolve contradictions in β-MCA’s role in cholesterol metabolism across species?
Advanced Research Focus
Discrepancies arise from species-specific bile acid pools (e.g., β-MCA is abundant in mice but absent in humans). To address this:
- Comparative studies : Use C57L mice (gallstone-susceptible) vs. Cyp2c70⁻/⁻ mice (human-like bile acid profile) to isolate β-MCA’s effects on biliary cholesterol saturation .
- Dietary controls : Pair β-MCA supplementation (0.5%) with lithogenic diets (2% cholesterol, 0.5% cholic acid) to evaluate gallstone prevention .
- Metabolomic profiling : Measure fecal bile acids via LC-MS to assess β-MCA’s microbial conversion to secondary bile acids (e.g., hyodeoxycholic acid) .
Q. How can researchers optimize chromatographic separation of β-MCA enantiomers for metabolic studies?
Methodological Focus
Separation of α- and β-MCA enantiomers requires reversed-phase HPLC with polarity-adjusted mobile phases:
- Column : C18 (150 mm × 2.1 mm, 3.5 μm), maintained at 40°C.
- Mobile phase : 0.1% formic acid with organic modifiers (e.g., acetonitrile:methanol mixtures) to modulate polarity components (α, β, π). A 70:30 aqueous:organic ratio achieves baseline separation with retention times <15 minutes .
- Detection : Electrospray ionization (ESI)-MS in negative ion mode for enhanced sensitivity .
Q. What are the critical considerations for designing in vivo studies on β-MCA’s impact on gut microbiota and metabolic health?
Advanced Research Focus
Key factors include:
- Dosing regimen : Oral gavage (10–50 mg/kg/day) vs. dietary supplementation (0.2–0.5% w/w) to mimic physiological exposure .
- Microbiota modulation : Use germ-free mice colonized with human fecal microbiota to assess β-MCA’s microbial metabolism .
- Endpoint analysis : Quantify conjugated forms (e.g., tauro-β-MCA) in portal blood and ileal content via LC-MS to evaluate enterohepatic cycling .
Q. How should researchers validate β-MCA’s purity and stability in experimental setups?
Basic Research Focus
- Purity assessment : HPLC with UV detection (λ = 210 nm) and ≥98% peak area threshold .
- Stability testing : Store β-MCA in methanol at –80°C to prevent degradation. For cell culture, dissolve in DMSO (≤0.1% final concentration) and confirm stability via LC-MS over 24 hours .
Q. What statistical approaches are recommended for analyzing β-MCA’s dose-dependent effects in metabolic studies?
Methodological Focus
- Nonlinear regression : Fit dose-response curves (e.g., β-MCA’s inhibition of FXR activity) using four-parameter logistic models.
- Multivariate analysis : Apply principal component analysis (PCA) to integrate metabolomic data (bile acids, lipids) and microbiome alpha-diversity indices .
- Power analysis : For in vivo studies, ensure n ≥ 8/group to detect a 30% change in biliary cholesterol saturation (α = 0.05, power = 80%) .
Q. How does β-MCA’s structure influence its solubility and bioavailability in experimental models?
Basic Research Focus
β-MCA’s low solubility in aqueous buffers (logP ≈ 1.5) necessitates micellar solubilization with tauro-conjugation or synthetic carriers (e.g., cyclodextrins) for in vitro assays. Bioavailability in mice is enhanced by co-administration with lipids (e.g., 10% olive oil) to facilitate intestinal absorption .
Propiedades
IUPAC Name |
(4R)-4-[(3R,5R,6S,7R,8S,9S,10R,13R,14S,17R)-3,6,7-trihydroxy-10,13-dimethyl-2,3,4,5,6,7,8,9,11,12,14,15,16,17-tetradecahydro-1H-cyclopenta[a]phenanthren-17-yl]pentanoic acid | |
---|---|---|
Source | PubChem | |
URL | https://pubchem.ncbi.nlm.nih.gov | |
Description | Data deposited in or computed by PubChem | |
InChI |
InChI=1S/C24H40O5/c1-13(4-7-19(26)27)15-5-6-16-20-17(9-11-23(15,16)2)24(3)10-8-14(25)12-18(24)21(28)22(20)29/h13-18,20-22,25,28-29H,4-12H2,1-3H3,(H,26,27)/t13-,14-,15-,16+,17+,18+,20+,21+,22-,23-,24-/m1/s1 | |
Source | PubChem | |
URL | https://pubchem.ncbi.nlm.nih.gov | |
Description | Data deposited in or computed by PubChem | |
InChI Key |
DKPMWHFRUGMUKF-CRKPLTDNSA-N | |
Source | PubChem | |
URL | https://pubchem.ncbi.nlm.nih.gov | |
Description | Data deposited in or computed by PubChem | |
Canonical SMILES |
CC(CCC(=O)O)C1CCC2C1(CCC3C2C(C(C4C3(CCC(C4)O)C)O)O)C | |
Source | PubChem | |
URL | https://pubchem.ncbi.nlm.nih.gov | |
Description | Data deposited in or computed by PubChem | |
Isomeric SMILES |
C[C@H](CCC(=O)O)[C@H]1CC[C@@H]2[C@@]1(CC[C@H]3[C@H]2[C@H]([C@H]([C@H]4[C@@]3(CC[C@H](C4)O)C)O)O)C | |
Source | PubChem | |
URL | https://pubchem.ncbi.nlm.nih.gov | |
Description | Data deposited in or computed by PubChem | |
Molecular Formula |
C24H40O5 | |
Source | PubChem | |
URL | https://pubchem.ncbi.nlm.nih.gov | |
Description | Data deposited in or computed by PubChem | |
Molecular Weight |
408.6 g/mol | |
Source | PubChem | |
URL | https://pubchem.ncbi.nlm.nih.gov | |
Description | Data deposited in or computed by PubChem | |
Physical Description |
Solid | |
Record name | 3a,6b,7b-Trihydroxy-5b-cholanoic acid | |
Source | Human Metabolome Database (HMDB) | |
URL | http://www.hmdb.ca/metabolites/HMDB0000415 | |
Description | The Human Metabolome Database (HMDB) is a freely available electronic database containing detailed information about small molecule metabolites found in the human body. | |
Explanation | HMDB is offered to the public as a freely available resource. Use and re-distribution of the data, in whole or in part, for commercial purposes requires explicit permission of the authors and explicit acknowledgment of the source material (HMDB) and the original publication (see the HMDB citing page). We ask that users who download significant portions of the database cite the HMDB paper in any resulting publications. | |
CAS No. |
2393-59-1 | |
Record name | β-Muricholic acid | |
Source | CAS Common Chemistry | |
URL | https://commonchemistry.cas.org/detail?cas_rn=2393-59-1 | |
Description | CAS Common Chemistry is an open community resource for accessing chemical information. Nearly 500,000 chemical substances from CAS REGISTRY cover areas of community interest, including common and frequently regulated chemicals, and those relevant to high school and undergraduate chemistry classes. This chemical information, curated by our expert scientists, is provided in alignment with our mission as a division of the American Chemical Society. | |
Explanation | The data from CAS Common Chemistry is provided under a CC-BY-NC 4.0 license, unless otherwise stated. | |
Record name | Cholan-24-oic acid, 3,6,7-trihydroxy-, (3alpha,5beta,6beta,7beta)- | |
Source | ChemIDplus | |
URL | https://pubchem.ncbi.nlm.nih.gov/substance/?source=chemidplus&sourceid=0002393591 | |
Description | ChemIDplus is a free, web search system that provides access to the structure and nomenclature authority files used for the identification of chemical substances cited in National Library of Medicine (NLM) databases, including the TOXNET system. | |
Record name | 3a,6b,7b-Trihydroxy-5b-cholanoic acid | |
Source | Human Metabolome Database (HMDB) | |
URL | http://www.hmdb.ca/metabolites/HMDB0000415 | |
Description | The Human Metabolome Database (HMDB) is a freely available electronic database containing detailed information about small molecule metabolites found in the human body. | |
Explanation | HMDB is offered to the public as a freely available resource. Use and re-distribution of the data, in whole or in part, for commercial purposes requires explicit permission of the authors and explicit acknowledgment of the source material (HMDB) and the original publication (see the HMDB citing page). We ask that users who download significant portions of the database cite the HMDB paper in any resulting publications. | |
Retrosynthesis Analysis
AI-Powered Synthesis Planning: Our tool employs the Template_relevance Pistachio, Template_relevance Bkms_metabolic, Template_relevance Pistachio_ringbreaker, Template_relevance Reaxys, Template_relevance Reaxys_biocatalysis model, leveraging a vast database of chemical reactions to predict feasible synthetic routes.
One-Step Synthesis Focus: Specifically designed for one-step synthesis, it provides concise and direct routes for your target compounds, streamlining the synthesis process.
Accurate Predictions: Utilizing the extensive PISTACHIO, BKMS_METABOLIC, PISTACHIO_RINGBREAKER, REAXYS, REAXYS_BIOCATALYSIS database, our tool offers high-accuracy predictions, reflecting the latest in chemical research and data.
Strategy Settings
Precursor scoring | Relevance Heuristic |
---|---|
Min. plausibility | 0.01 |
Model | Template_relevance |
Template Set | Pistachio/Bkms_metabolic/Pistachio_ringbreaker/Reaxys/Reaxys_biocatalysis |
Top-N result to add to graph | 6 |
Feasible Synthetic Routes
Descargo de responsabilidad e información sobre productos de investigación in vitro
Tenga en cuenta que todos los artículos e información de productos presentados en BenchChem están destinados únicamente con fines informativos. Los productos disponibles para la compra en BenchChem están diseñados específicamente para estudios in vitro, que se realizan fuera de organismos vivos. Los estudios in vitro, derivados del término latino "in vidrio", involucran experimentos realizados en entornos de laboratorio controlados utilizando células o tejidos. Es importante tener en cuenta que estos productos no se clasifican como medicamentos y no han recibido la aprobación de la FDA para la prevención, tratamiento o cura de ninguna condición médica, dolencia o enfermedad. Debemos enfatizar que cualquier forma de introducción corporal de estos productos en humanos o animales está estrictamente prohibida por ley. Es esencial adherirse a estas pautas para garantizar el cumplimiento de los estándares legales y éticos en la investigación y experimentación.